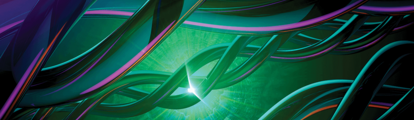
Engineering Non-Invasive Refractive Correction
Could femtosecond laser-induced corneal crosslinking transform standard refractive surgery – and provide a vision correction procedure that is suitable for all?
At a Glance
- Normal femtosecond laser surgery employs a highly-focused beam, resulting in a high-density plasma that creates pressure waves, which can disrupt corneal biomechanics and cause post-surgical complications
- By contrast, a loosely-focused femtosecond beam creates a low density plasma (LDP) that does not generate damaging pressure waves
- We have discovered that LDP, by ionizing water and creating oxygen free radicals, creates crosslinks in corneal proteins and thereby alters corneal geometry in a modulatable, predictable way
- Results from animal models suggest that our LDP approach could be the basis of safer, non-invasive, permanent vision correction procedures suitable for virtually all patients.
My laboratory in the Department of Mechanical Engineering at Columbia University (New York, USA) approaches ophthalmology in a very different way from most: in particular, our expertise in biomechanics and laser materials processing provides a fresh perspective on the eye. Of course, we’ve had to learn a lot about collagen and tissue biomechanics – very different from the single crystal and bicrystal mechanics that we’ve previously worked on – but good things happen when you embrace new concepts and think outside the box. In fact, our open-minded approach helped us make a key discovery: a loosely-focused laser beam can non-invasively modulate corneal geometry and stably alter the eye’s refractive power.
Clouds not avalanches
I am a mechanical engineer by training, and have always been fascinated by the plasma physics of ultrafast lasers. In retrospect, this turned out to be the perfect background to kick-start our investigations into the adjustment of corneal biomechanics. Nevertheless, making progress in this field required a great deal of time and effort; for example, once we’d found that a loosely-focused laser beam strengthened the cornea, we had to go back to first principles and do some very basic science to figure out what was happening. It took years! The end result is a procedure that is rather different from the standard ophthalmological application of lasers – we are using femtosecond lasers not to cut tissue, but to strengthen it by inducing crosslinks.
How does this work? Normally, surgical use of femtosecond lasers relies on ‘avalanche ionization,’ where multiple photons ionize a substrate molecule; the freed electrons hit electrons associated with other molecules, thereby ionizing other molecules and liberating more electrons, and so on. The cascade effect requires the electron cloud to reach a critical density such that all the incoming photons from the laser pulse are absorbed by electrons, thus creating a dense plasma. Being under pressure, however, the plasma expands and creates damaging shock waves and cavitation bubbles. Hence, normal femtosecond laser operation allows the surgeon to cut a flap in the cornea but also causes unwanted damage, and may lead to post-surgical complications, such ectasia and corneal weakening.
By contrast, our approach involves modulating the power and focus of the laser beam such that there are enough photons to create an ionization cloud, but not enough for avalanche ionization and dense plasma formation. The resulting low density plasma (LDP) does not generate shockwaves, and therefore does not damage biological tissue in the same way as a high density plasma. Importantly, however, the LDP ionizes water molecules, thereby generating oxygen free radicals which react with corneal proteins, creating crosslinks that strengthen the cornea in the precise area covered by the laser. The ability to target crosslink induction to very small volumes of corneal tissue allows us to precisely and predictably modulate corneal geometry.
Correction without cutting
Once we had shown that we were creating crosslinks that strengthened the cornea, I knew we’d found something important: a new therapeutic approach that could be tailored to different patients’ needs. Our next step was to conduct proof-of-principle studies in enucleated porcine eyes and in rabbit eyes in vivo (see ‘Plasma physics for configuring corneas’). For the in vitro porcine eye studies, we removed the epithelial layer, not because our approach requires it, but because abattoir-sourced eyes often have superficial damage that can interfere with imaging. We didn’t need to remove the epithelial layer in the rabbit animal models. Indeed, a big advantage of our method is that it is truly non-invasive.
The results from these animal studies are highly promising. In enucleated porcine eyes, LDP-mediated corneal flattening (as would be used for the treatment of myopia) changed refractive power by about 12 percent (~ 5.11 diopters) during the initial 8 hours. After 24 hours of recovery, refractive power stabilized at ~92 percent (~3.45 diopters) of the initial level. In LDP-mediated corneal steepening (as would be used for the treatment of hyperopia), the refractive power of enucleated porcine eyes increased gradually for 12 hours before stabilizing. In no case was there evidence of laser-induced thermal denaturation or other tissue damage. Additionally, we have very new and exciting – albeit preliminary – observations regarding LDP treatment and keratinocyte populations.
In rabbit eyes in vivo, we observed a relative change in effective refractive power of ~1.94 diopters for treated eyes (the difference between porcine and rabbit eyes in the refractive change achieved is expected, as the two species have very different corneal geometries). Treatment of live rabbits was not associated with the wounds or wound-healing responses seen in refractive surgery. Equally, there was no evidence of thermal damage, such as edema or endothelial cell detachment; microscopy after sacrifice suggested that treated eyes were no different from controls. Some of our treated rabbits have now been followed up for seven months; data suggest that the refractory power change is stable, and confocal imaging indicates that the eyes show no significant structural changes over this period. And that’s exactly what we expected; remember that we are creating covalent bonds, which are difficult to break. Therefore, as long as there is no significant collagen turnover, the refractive power changes we achieve should persist.
We are very pleased with our data so far, but we’d also be delighted to work with anyone who wants to try replicating our results; we all have a duty to make sure that our data are valid. After all, we want to develop a treatment that works every time – 85 or 90 percent success rates are not good enough. Excellent outcomes, every time: that’s our goal.
Future focus
Currently, we are moving ahead in two major directions: basic research and clinical development. The former will include extensive parametric studies to ensure that we know exactly what is going on during LDP treatment of the cornea. And however many samples the power analysis tells me I should do, I will always multiply that by five! It upsets the statisticians, but my view is that you only need to mess up once to lose credibility. In addition, we are developing a model that treats crosslinks as external load, and developing equations relevant to this; the aim is to fully understand the cornea’s viscoelastic response to the LDP procedure. This knowledge should enable us to precisely tailor LDP treatment to each patient. Treating crosslinks as a load has not been done before, at least not to my knowledge – so there’s enough work for two PhD theses! We are also looking at longitudinal studies on the nature of the crosslinks generated by LDP.
With regard to clinical trials, we are working hard on developing suitable device prototypes and procedures. I’d hope to be in human trials by the end of the year, certainly some time in 2019. We are already talking with potential partners about clinical trials in Europe – people have been very enthusiastic whenever we present this work. In particular, we are optimizing the procedure so that it is at least on a par with LASIK with regard to treatment time; everyone would prefer a 5 minute procedure to a 40 minute procedure. We also intend to modify the LDP procedure so that it is applicable to other conditions, including keratoconus, which is the condition we were originally targeting before our ophthalmological colleagues steered us towards refractive surgery. In fact, keratoconus is a great opportunity, given that the current standard of care requires ‘epithelium-off’ crosslinking. Our ‘epi-on’ approach would remove many risks, and could represent a significant advance.
We are also looking at applications beyond ophthalmology; for example, applying LDP to articular cartilage in osteoarthritis has given us some fantastic results, and we have now received NIH grants to further pursue this.
Plasma physics for configuring corneas
Porcine eyes:
- Fresh eyes (~2 hours old; n=60) were mounted in a chamber that permits control of IOP
- Epithelia were removed because of abattoir-associated damage that would interfere with imaging
- A coverslip was applied to press the cornea into a single plane and thereby allow consistent delivery of laser energy over the treated area
- The laser was applied in a boustrophedon pattern; successively deeper ‘treatment layers’ were achieved by consecutive applications at increasing depth (50 µm increments)
- Treatment of square 5 mm by 5 mm, over the center of the eye, induced corneal flattening (n=15; matched with 10 controls)
- Treatment of ring-shaped area induced corneal steepening (n=13; matched with 10 controls)
- A separate control study was undertaken to evaluate any effects of experimental set-up itself (n=12)
- After treatment, refractive power was measured hourly over 24 hours
- After 24 hours, corneas were cultured for one week to assess stability of crosslinking
Rabbit eyes:
- Mainly as above, but modified to accommodate live animal (for example, the coverslip arrangement permitted addition of drops to keep eyes moist)
- Epithelium left in place
- Treated area of 5 mm diameter
- Followed up at 24 hours, 7 days, then weekly up to 3 months
Clear benefits
The theoretical advantages are profound. Firstly, being non-invasive, our technique is preferable to standard surgical approaches to vision correction. Furthermore, our approach is gentler than other crosslinking methods: we do not need to peel off the keratocytes, and we don’t need to risk damage to keratocytes and epithelial cells by applying UV light, as required in standard crosslinking. Secondly, unlike standard surgical procedures, our method will be applicable to ‘difficult’ patients; for example, older individuals, patients with thin corneas, people with dry eye syndrome, and those with abnormal corneas. Thus, we hope that many people who are currently ineligible for refractive surgery could be treatable by our method.
I firmly believe that LDP could be the start of a new standard of care in permanent vision correction.
Sinisa Vukelic is a Lecturer in Discipline at Columbia University, New York, NY, USA. His laser research interests lie in laser induced mechanical deformation, single crystal and bicrystal micromechanics and strain gradient plasticity, structural modification of transparent dielectrics and laser imaging and diagnostics.
Illustrations by Andy Potts for Vukelic Group and Clarity Vision Technologies.
- C Wang, et al., “Femtosecond laser crosslinking of the cornea for non-invasive vision correction”, Nature Photonics, doi.org/10.1038/s41566-018-0174-8.
Sinisa Vukelic is a Lecturer in Discipline at Columbia University, New York, NY, USA. His laser research interests lie in laser induced mechanical deformation, single crystal and bicrystal micromechanics and strain gradient plasticity, structural modification of transparent dielectrics and laser imaging and diagnostics.