With Akishi Onishi, Senior Scientist at the Laboratory for Retinal Regeneration, RIKEN Center for Developmental Biology
Kenji Imoto, Global Development Lead, Gene Therapy, Santen
Abu Abraham, VP, Vitreous & Retina TA Strategy, Santen
Yatish Lad, Head of Early Development, Oxford Biomedica
Jason Slingsby, Chief Business Officer, Oxford Biomedica
Kyriacos Mitrophanous, Chief Scientific Officer, Oxford Biomedica
Today, genomic data have a central role in medicine. Genetic tests support patient care at multiple points from diagnosis to clinical management. Each year, we’re more confident about pinpointing the patients who will best respond to a particular therapy – and why. More personalized, more effective approaches are here to stay.
At the same time, regenerative medicine such as gene therapy has at last come of age. Key advances include development of genetic delivery mechanisms that mediate safer, more stable expression; in particular, vectors derived from adeno-associated virus (AAV) and lentivirus are associated with fewer adverse effects and less express shut-down that plagued earlier approaches (1, 2). Indeed, one of the most successful gene therapy approaches to date – the CAR T ex vivo system (3, 4) – relies on lentiviral vectors.
Ophthalmology is at the forefront of this sea-change in patient care. The first FDA approval of a gene therapy for a genetic disease – voretigene neparvovec – was for an ocular indication (RPE65 mutation-associated retinal dystrophies) causing vision loss that can result in blindness (5). This development will have consequences beyond RPE65 disease; a rising tide lifts all boats, and other ocular gene therapies poised to sail into the market include those targeting geographic atrophy, uveitis, some corneal dystrophies and neovascular age-related muscular degeneration (AMD). Clearly, tomorrow’s ophthalmologists will navigate very different courses from those who are restricted by today’s therapies.
Inherited retinal disorders (IRDs) have long been considered the most promising targets for ocular gene therapy. Firstly, as Abu Abraham, VP, Vitreous & Retina TA Strategy at Santen, says, these diseases represent a significant unmet medical need; the majority of IRDs inevitably lead to severe vision loss or blindness and disproportionally affect children and young adults (6, 7, 8). Jason Slingsby, Chief Business Officer from Oxford Biomedica also highlights that currently many IRDs have no effective treatment; the current standard of care (such as tinted lenses to reduce illumination of the retina) can only slow the rate of photoreceptor degeneration (9). This justifies investment in complex treatment strategies such as gene therapy, particularly given that the causative mutations have already been defined in many IRDs. Furthermore, IRDs that are monogenic may be the most amenable to respond to gene therapies (2).
Secondly, IRD gene therapy is expected to be relatively safe: as an immune-privileged tissue, the retina should not develop a significant inflammatory response to components of the gene therapy. The ocular anatomy further favors gene therapy approaches: the eye can be monitored non-invasively after treatment, thereby simplifying clinical assessments of disease progression and therapeutic response (2, 10, 11, 12, 13).
Finally, the target tissue for an IRD gene therapy – the retina – is small; the vector quantities required for a therapeutic effect are therefore relatively small, with obvious implications for economics. Better still, the retina is relatively accessible, which simplifies administration of gene therapy. Standard routes include intravitreal and subretinal injection; of these, the subretinal route is more precise and efficient. Intraocular inflammation and cataract are more common with intravitreal injection, while retinal detachment is a risk with both intravitreal and subretinal injection. Ocular hemorrhage is more common with subretinal injection due to the surgical procedure required. Accordingly, subretinal administration methods and new techniques are being optimized and explored, respectively (10, 11, 12, 13, 14).
Drug development is rarely simple, and gene therapies have the potential to be particularly complex. As Abraham notes, gene therapy development involves a broad range of challenges, from vector construction and titer optimization to efficient transduction of target cells. “Even after you have a finished product, you have to pay attention to factors such as administration,” he says. “The efficiency of subretinal delivery is related to the precision of the surgeon.”
Before gene therapy development begins, there is the need to achieve a more complete understanding of the genetic basis for IRDs. While progress has been made in identifying the genetic causes of many IRDs, there are still gaps in knowledge. As Kenji Imoto, Global Development Lead, Gene Therapy, Santen, points out, the causative mutations remain unknown in approximately half of all patients (9). “You can’t design a gene therapy without identifying the causative gene,” he says. And elucidating IRD genetics is only the first step; we then need to construct vectors that mediate expression of a corrective nucleic acid sequence at sufficient levels, over sufficient periods of time and with sufficient safety.
This kind of vector construction raises its own challenges; if the causative gene is large, or if multiple genes are affected, the vector will need to accommodate relatively long sequences of therapeutic DNA, for example. Consider IRDs such as Stargardt’s, Usher IB, Leber congenital amaurosis-10 and retinitis pigmentosa (15) – most vectors cannot hold transgenes of the size required to treat these conditions. Options include adopting a mini-gene approach or splitting the therapeutic gene; unfortunately, these approaches are less efficient than using an intact transgene. Fortunately, lentiviral vectors have a cargo capacity sufficient for many of the larger transgenes, and can even accommodate multiple transgenes (16).
An additional challenge of working with large transgenes, however, is that they are associated with sub-optimal vector titers. But again, the industry has risen to the challenge: in particular, Oxford Biomedica has improved vector titers through a combination of design of experiments (DoE) optimization, development of suspension culture methods for vector manufacture, and other proprietary innovations. Jason Slingsby says gene-based correction for life-long conditions, such as IRDs, requires a technology platform capable of mediating long-term efficacy (stable gene expression) with acceptable safety. To protect sight in these patients, and to avoid the huge impact of vision loss on them and their families, fundamentally new therapeutic approaches are required. And Abraham predicts the introduction of other new technologies, such as non-viral plasmids, will further optimize vector titers.
We should also remember that not all genetic diseases are amenable to correction via provision of a normal protein by gene transfer. “The standard gene therapy approach won’t be effective in cases where the disease is caused by the presence of an abnormal protein rather than by the absence of a normal protein,” says Imoto. In such cases – as in RHO gene mutation – gene therapy will require access to complementary expertise and intellectual property, he says; for example, in the field of gene editing (17).
Other issues in the field of IRD gene therapy include difficulties in demonstrating the safety and efficacy of therapies aimed at this group of diseases. In particular, the clinical course of many IRDs is imperfectly understood – and this complicates the assessment of new treatments. However, for orphan conditions, such as IRDs, regulatory bodies such as the FDA may support natural history studies aimed at collecting information about the course of disease, as does the International Rare Disease Research Consortium (18, 19). “Natural history studies must be rigorously designed and executed, but they can provide information that is of enormous value when designing a clinical trial for a little-understood condition,” says Abraham.
Similarly, many IRDs are associated with very small patient populations; Imoto notes how this reality may impede demonstration of a statistically significant effect in clinical trials. For this reason, says Abraham, the FDA has relaxed its usual requirement for a drug to show statistically significant efficacy in two clinical trials before it can be approved: “For some IRDs,” he says, “only one study is required, because of the rarity of the disease.” The EMA also recognizes that distinctive characteristics and features of gene therapies have an impact on product development (20). Japanese regulators may “conditionally approve” a new drug if sponsors demonstrate early efficacy (prior to completion of Phase III trials). Such rapid approval system allows manufacturers to market the drug for up to seven years while continuing its formal development in parallel (21). “These kinds of regulatory moves really help companies accelerate the development of orphan therapies,” says Abraham. Even so, to collect sufficient clinical data, companies may consider international cooperation. Not only can this assist patient recruitment generally, but also, as Imoto reminds us, in some IRDs the causative genes are associated with regional differences in mutation frequency (9, 22). “For such diseases, it may be appropriate to focus clinical development in specific geographic regions,” he says. Moreover, adds Abraham, locating clinical trials in non-Western territories may give rise to other advantages, including cost savings.
To further combat the challenges associated with clinical trials, the Riken Institute in Kobe has developed retinal organoids, which mimic human retinogenesis and are used as clinical tools to model new treatments, such as gene therapies [See Box Retinal Organoids]. The retina is made up of the neural retina and the retinal pigment epithelial (RPE) layer. Since the RPE consists of a single layer of retinal pigment epithelial cells, researchers can reproduce the RPE layer by isolating and expanding differentiated RPE cells on a culture dish (23). Akishi Onishi, Senior Scientist at the Laboratory for Retinal Regeneration, RIKEN Center for Developmental Biology explains: “We supplement chemical compounds and proteins that induce retinal differentiation, and retinal tissue emerges from the aggregates. When we use human embryonic stem (ES)/ induced pluripotent stem (iPS) cells, the layered structure of the retina can be seen after approximately one month. Retinal organoids allow us to obtain retinal neurons that are comparable to those of native retinas.” Onishi talks about his inspiration for studying retinal organoids – and the development of the system: “Over the course of my research, I have been studying molecular functions involved in retinal development and physiology. A few years ago, I was profoundly impressed by a report on successful production of retinal organoids from human embryonic stem cells (24), which was really encouraging.”
Finally, bringing gene therapy to market also requires attention to post-development issues. “Drug prices and payment systems represent a major barrier,” says Imoto, noting how difficult it is for drug companies to recoup development costs for therapies that are administered infrequently or as one-off treatments. “And that contributes to high drug prices and complicates reimbursement – which can limit accessibility for many patients.”
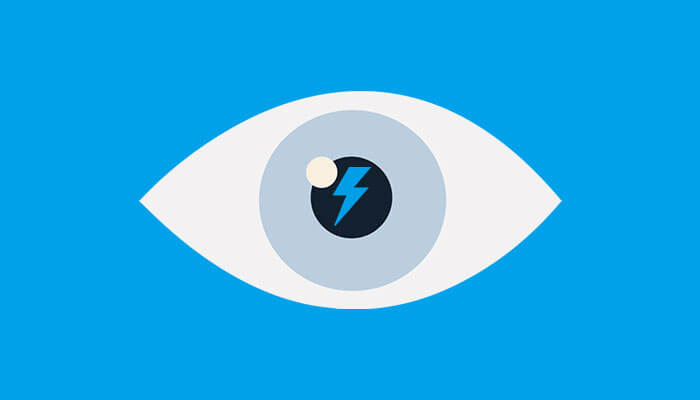
The range of expertise needed – and the level of financial resources required – suggest that bringing an IRD gene therapy to market would be a challenge for any single organization. It requires co-operation between multiple stakeholders, including patients, advocacy groups, ophthalmologists, biotech, pharma and academia, which is why Santen and Oxford Biomedica entered into an R&D collaboration in June 2019. Unusually, this collaboration brings synergies at all stages of the product life cycle, from pre-clinical development to health economics. In the development, manufacturing and post-marketing stages, Santen accesses the advantages of Oxford Biomedica’s lentiviral vector technology [See LentiVector® - A third-generation vector] and associated manufacturing and proprietary know how. John Dawson, Chief Executive Officer at Oxford Biomedica, adds: “We have been working on IRD gene therapy for over 15 years and now teaming up with Santen to accelerate clinical translation of our novel approach.” Oxford Biomedica benefits from Santen’s long-established R&D links to the Riken Institute, thereby gaining access to Riken’s world-leading retinal organoid models [See Box Retinal Organoids].
Describing the potential of retinal organoids, Onishi says his team can now use retinal tissues that are closer to native retinas as experimental materials. He notes, “This likeness implies to both ‘quality’ and ‘quantity’, allowing us to obtain retinal neurons with a high degree of certainty of differentiation in the same number (percentage) as in vivo. Also, we can conduct drug screening studies with the organoids and/or specific retinal neurons isolated from the organoids, such as retinal ganglion cells.” In addition, since the formation process of retinal organoids is almost comparable to in vivo differentiating retinas, it is useful for disease modeling of a number of retinal diseases. (25) “These models may be key in developing a novel gene therapy for the IRD in question,” asserted Yatish Lad, Head of Early Development, Oxford Biomedica.
For Abraham, gene therapy’s potential for patient benefit is unarguable: “It’s a new way to treat diseases that were previously untreatable – and it’s applicable to a broad range of IRDs.” He adds that gene therapy is not limited to delivery of corrective genes or knock-out sequences for treatment of genetic disease. “We can also use it to mediate more conventional therapeutic approaches such as anti-VEGF therapy.”
In addition to gene therapies, Santen has also entered into a partnership with jCyte, a clinical stage biotechnology company focused on degenerative retinal diseases, to develop and launch an investigational cell therapy to treat retinitis pigmentosa, which affects approximately 1.9 million people worldwide and 3.7 in 10,000 Europeans (26, 27).
- LentiVector® comprises a broad range of patents and know-how; at its core is a third-generation replication-incompetent vector that has been designed with patient safety in mind from the outset (29, 30)
- It accommodates large transgenes or even multiple transgenes – which is particularly attractive in the context of IRD gene therapy, as in many cases the genes involved are too large to fit into other vectors (29, 31)
- Genomic insertion is targeted to lentiviral integration sites within open reading frames, which favors long-term transgene expression (28)
- LentiVector®-mediated gene therapy may correct a genetic defect throughout the lifetime of a transduced cell (29)
- A single sub-retinal administration of a LentiVector® has been shown to mediate long-term (up to 4.5 years) expression of endostatin and angiostatin proteins in AMD patients (n=21) (30)
Over the next few years, Abu Abraham expects Santen to have a clinical gene therapy program for inherited retinal disorders, reaching clinical stage within two to three years. Abraham would like to see agencies worldwide working together to facilitate an accelerated approval process, developing a viable option for patients affected by these diseases.
As Oxford Biomedica experts comment, in the last few years several gene and cell therapies have been approved, including Luxturna for the IRD LCA2 (RPE65 deficiency) (4), and many more are in preclinical and clinical development. Many of these programs are for patients with IRDs, so the expectation is that in the coming years many more therapies will become available. They explain: “The reality of IRD on patients and their families are a key motivator for our scientists; we believe that gene therapy could become a key modality for treatment. Both of these criteria are met by Oxford Biomedica’s LentiVector® technology.”
Akishi Onishi says retinal organoids are now widely used in drug discovery and regenerative medicine research, as well as in evaluating the safety and efficacy of gene therapies. In the near future, it is expected that retinal organoid techniques will become more sophisticated, with more in vivo-like properties – and that will make these studies more efficient, as well as being useful for disease-modeling pathological studies of photoreceptor degeneration diseases, such as retinitis pigmentosa. Onishi considers the possibilities for a wider use of retinal organoids: “I predict that clinicians will be able to provide a more accurate treatment by the use of these models. When patient-derived retinal organoids are available in the future, it might be possible to phenocopy patients’ retinal disease in vitro as well as in vivo.” He predicts that clinicians would be able to try medicines that protect the disease phenotype with the use of retinal organoids, as well as estimate the optimal dosage of the drug. Also, if a genetic diagnosis identifies the causative gene, it is likely that the effect of the gene therapy formulation of the causative gene can be tested using this method. Retinal organoids are a beautiful experimental system, but there are barriers that must be overcome before this model is adopted for wider use.
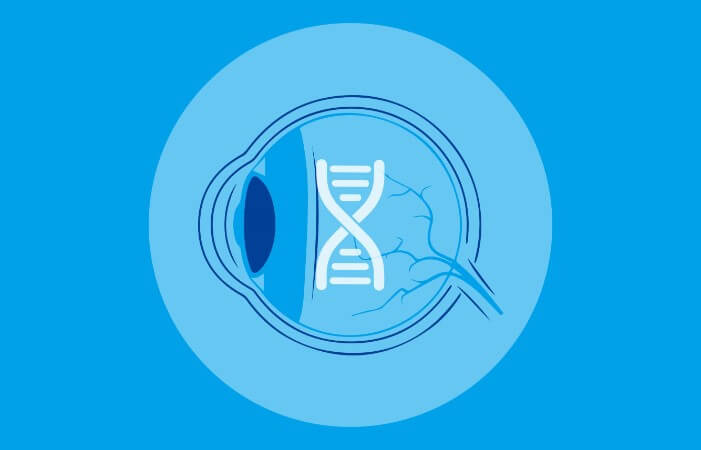
Retinal organoids, which form organized layered retinal structures displaying markers for typical retinal cell types, mimicking human retinogenesis, are used as clinical tools to model new treatments, such as gene therapies (32, 33, 34).
- Cost-effective, rapid means of assessing whether the vector can mediate therapeutic transgene expression in photoreceptor cells prior to costly animal studies (35)
- Permits assessment of vector-mediated gene expression in a human cell system which has the mutation of interest (not all animals will express the gene or exhibit the mutation associated with human disease) (36)
- Permits assessment of vector targeting to the cells and cell compartments of interest (36)
- Excellent resource for early non-clinical studies; guides subsequent development (37).
Important considerations
1. Retinal organoids are not identical to the native retina, even though the retinal organoids produce a three-layered structure (38)
- In human retinal organoids, photoreceptor cells are located on the surface and the ganglion cells on the inside. The organoid photoreceptor cells have a shorter outer segment structure and have difficulty creating a macula region using current technology (39). Also, there is no axon outgrowth from organoid retinal ganglion cells and there is no structure in the optic nerve head where the ganglion cell axons elongate, without external signalling cues (40, 41)
2. Retinal organoids develop at almost the same rate as native retinas, so they are appropriate to study retinal diseases in the early developmental stage. However, they do not appear to form normally during later stages of development, which could cause difficulties in analyzing the pathogeneiss of age-related retinal diseases as these need to be cultured for a long time (42, 43)
3. The quality of retinal organoid differentiation depends on the ES/IPS cells used as evidence has shown these cells to exhibit high variation in terms of quality (44, 45)
- Researchers often establish multiple lines of ES/iPS cells from a single donor sample. The quality differs, resulting in differences in the success rate of differentiation and developmental maturation (46).
References
- C Li, RJ Samulski, “Engineering adeno-associated virus vectors for gene therapy,” Nature Reviews Genetics, 21, 4 (2020). PMID: 32042148.
- C Bostan, CX Qian, “Gene therapies for inherited diseases,” Review of Ophthalmology (2019). Available at: https://bit.ly/2MjkBOm.
- S Ghassemi et al., “Reducing ex vivo culture improves the antileukemic activity of chimeric antigen receptor (CAR) T cells,” Cancer Immunol Res, 6, 1100 (2018). PMID: 30030295.
- CW Freyer, “Tisagenlecleucel: the first CAR on the highway to remission for acute lymphoblastic leukemia,” J Adv Pract Oncol, 9, 537 (2018). PMID: 31086690.
- US Food & Drug Administration, “FDA approves novel gene therapy to treat patients with a rare form of inherited vision loss” (2017). Available at: https://bit.ly/2VMV4S2.
- J Smith et al., “New and emerging technologies for the treatment of inherited retinal diseases: a horizon scanning review,” Eye, 29, 1131 (2015). PMID: 26113499.
- O Galvin et al., “The impact of inherited retinal diseases in the Republic of Ireland (ROI) and the United Kingdom (UK) from a cost-of-illness perspective,” Clin Ophthalmol, 14, 707 (2020). PMID: 32184557.
- JL Duncan et al., “Inherited retinal degenerations: current landscape and knowledge gaps,” Transl Vis Sci Technol, 7, 6 (2018). PMID: 30034950.
- JA Sahel et al., “Clinical characteristics and current therapies for inherited retinal degenerations,” Cold Spring Harb Perspect Med, 5 (2015). PMID: 25324231.
- L Petit et al., “Advances in gene therapy for diseases of the eye,” Hum Gen Ther, 27, 563 (2016). PMID: 27178388.
- L Ziccardi et al., “Gene therapy in retinal dystrophies,” Int J Mol Sci, 20, 5722 (2019). PMID: 31739639.
- I Trapani, A Auricchio, “Has retinal gene therapy come of age? From bench to bedside and back to bench,” Hum Mol Genet, 28, R108 (2019). PMID: 31238338.
- K Willett, J Bennett, “Immunology of AAV-mediated gene transfer in the eye,” Front Immunol, 4 (2013). PMID: 24009613.
- W Bethke, “The Future of Gene Therapy,” Review of Ophthalmology (2016). Available at: https://bit.ly/2TZQpvN.
- V Cavalieri et al., “Non-primate lentiviral vectors and their applications in gene therapy for ocular disorders,” Viruses, 10, 316 (2018). PMID: 29890733.
- I Trapani, A Auricchio, “Seeing the light after 25 years of retinal gene therapy,” Trends Mol Med, 24, 669 (2018). PMID: 29983335.
- M Diakatou et al., “Genome editing as a treatment for the most prevalent causative genes of autosomal dominant retinitis pigmentosa,” Int J Mol Sci, 20, 2542 (2019). PMID: 31126147.
- FDA, “Rare diseases: Natural history studies for drug development guidance for industry” (2019). Available at: https://bit.ly/2XUgHkn.
- S Day, “Recommendations for the design of small population clinical trials,” Orphanet J Rare Dis, 13, 195 (2018). PMID: 30400970.
- European Medicines Agency, “Guideline on quality, non-clinical and clinical requirements for investigational advanced therapy medicinal products in clinical trials” (2019). Available at: https://bit.ly/3dslGPW.
- Japan Pharmaceutical Manufacturers Association, “Pharmaceutical Administration and Regulations in Japan” (2018). Available at: https://bit.ly/2U2wQmN.
- Y Koyanagi et al., “Genetic Characteristics of retinitis pigmentosa in 1204 Japanese patients”, J Med Genet, 56, 662 (2019). PMID: 31213501.
- JP Heller et al., “A method for the isolation and culture of adult rat retinal pigment epithelial (RPE) cells to study retinal diseases”, Front Cell Neurosci, 9, 449 (2015). PMID: 26635529.
- T Nakano et al., “Self-formation of optic cups and storable stratified neural retina from human ESCs”, Cell Stem Cell, 10, 771 (2012). PMID: 22704518.
- S.I Ito et al., “Chemically-induced photoreceptor degeneration and protection in mouse iPSC-derived three-dimensional retinal organoids,” Stem Cell Res, 24, 94 (2017). PMID: 28886418.
- European Medicines Agency, “Retinitis pigmentosa” (2017). Available at: https://bit.ly/3johDaJ.
- C Hamel, “Retinitis pigmentosa,” Orphanet Journal of Rare Diseases, 1, 40 (2006). PMID: 17032466.
- CV Hacker et al., “The integration profile of EIAV-based vectors,” Mol Ther, 14, 536 (2006). PMID: 16950499.
- K Binley et al., “Transduction of photoreceptors with equine infectious anemia virus lentiviral vectors: safety and biodistribution of StarGen for Stargardt disease,” Invest Ophthalmol Vis Sci, 54, 4061 (2013). PMID: 23620430.
- PA Campochiaro et al., “Lentiviral vector gene transfer of endostatin/angiostatin for macular degeneration (GEM) study,” Hum Gen Ther, 28, 99 (2017). PMID: 27710144.
- M Azzouz et al., “Multicistronic lentiviral vector-mediated striatal gene transfer of aromatic L-amino acid decarboxylase, tyrosine hydroxylaser, and GTP cyclohydrolase I induces sustained transgene expression, dopamine production, and functional improvement in a rat model of Parkinson’s disease,” J Neurosci, 22, 10302 (2002). PMID: 12451130.
- M Phillips et al., “Modeling human retinal development with patient-specific induced pluripotent stem cells reveals multiple roles for visual system homeobox 2,” Stem Cells. 32, 1480 (2014). PMID: 24532057.
- A Buskin et al., “Disrupted alternative splicing for genes implicated in splicing and ciliogenesis causes PRPF31 retinitis pigmentosa,” Nat. Commun, 9, 1 (2018). PMID: 30315276.
- A Lane et al., “Modeling and Rescue of RP2 Retinitis Pigmentosa Using iPSC-Derived Retinal Organoids,” Stem Cell Reports, 15, 67 (2020). PMID: 32531192.
- M Garita-Hernandez et al., “AAV-mediated gene delivery to 3D retinal organoids derived from human induced pluripotent stem cells,” Int J Mol Sci, 3, 21, (2020). PMID: 32028585.
- P Ovando-Roche et al., “Harnessing the Potential of Human Pluripotent Stem Cells and Gene Editing for the Treatment of Retinal Degeneration,” Current Stem Cell Reports, 3, 112 (2017). PMID: 28596937.
- A Sridhar et al., “Single-Cell Transcriptomic Comparison of Human Fetal Retina, hPSC-Derived Retinal Organoids, and Long-Term Retinal Cultures,” Cell Rep, 30, 1644 (2020). PMID: 32023475.
- CB Mellough et al., “Systemic comparison of retinal organoid differentiation from human pluripotent stem cells reveals stage specific, cell line, and methodological differences,” Stem Cells Transl Med, 8, 694 (2019). PMID: 30916455.
- K Kruczek, A Swaroop, “Pluripotent stem cell-derived retinal organoids for disease modeling and development of therapies,” Stem Cells, [Epub ahead of print] (2020). PMID: 32506758.
- CM Fligor et al., “Three-dimensional retinal organoids facilitate the investigation of retinal ganglion cell development, organization and neurite outgrowth from human pluripotent stem cells,” Sci Rep, 8 (2018). PMID: 30266927.
- W Kobayashi et al., “Culture systems of dissociated mouse and human pluripotent stem cell-derived retinal ganglion cells purified by two-step immunopanning,” Invest Ophthalmol Vis Sci, 59, 776 (2018). PMID: 29392326.
- K. J Wahlin et al., “Photoreceptor outer segment-like structures in long-term 3D retinas from human pluripotent stem cells,” Sci Rep, 7, 766 (2017). PMID: 28396597.
- X Zhong et al., “Generation of three-dimensional retinal tissue with functional photoreceptors from human iPSCs,” Nat Commun, 5, 4047 (2014). PMID: 24915161.
- T Wakui et al., “Method for evaluation of human induced pluripotent stem cell quality using image analysis based on the biological morphology of cells,” J Med Imaging, 4, 044003 (2017). PMID: 29134187.
- Y Buganim et al., “The developmental potential of iPSCs is greatly influenced by reprogramming factor selection,” Cell Stem Cell, 15, 295 (2014). PMID: 25192464.
- G Liang & Y Zhang, “Genetic and epigenetic variations in iPSCs: Potential causes and implications for application,” Cell Stem Cell, 13, 149 (2013). PMID: 23910082.