VEGF has Physiolgical as well as Pathological Functions
Growing evidence for the key role played by VEGF in the normal adult eye now demands that ophthalmologists give careful consideration to the use of anti-VEGF therapies. While these agents are highly effective for the treatment of ocular neovascularization – particularly as an anti-permeability factor – it may also interfere with the normal functions of VEGF in the eye.
Vascular endothelial growth factor (VEGF, also known as VEGFA) was originally identified in 1989, as a protein that could stimulate the proliferation of vascular endothelial cells (1). The earliest VEGF studies focused on the fact that it appeared to be involved in the induction and maintenance of tumor vascularization. At around the same time, another research group, spurred by the observation that tumor blood vessels are excessively leaky, discovered a protein that could induce vascular permeability, and called it vascular permeability factor (2). In fact, most new blood vessels – with the apparent exception of those formed during development – are particularly permeable. The gene sequences of both molecules were determined in 1989, and it was discovered that the proteins that mediated these two activities – angiogenesis (capillary growth) and permeability – were actually the same, and ever since it has been referred to as VEGF (3).
Later, VEGF was found to be critical for vascular development. Its genetic deletion – even the deletion of one allele – from mice was catastrophic. It resulted in very early embryonic lethality, characterized by the absence of yolk sac vasculature and failure to form an aorta. Other work demonstrated that VEGF was involved in wound healing and the normal angiogenesis associated with reproduction – such as in the formation of the corpus luteum and the placenta. Blockade of VEGF, or of its primary signaling receptor VEGFR2, was shown in experimental models to suppress tumor growth. This observation stimulated great excitement regarding the possibility of a “magic bullet” that could inhibit primary tumor growth as well as prevent the formation of metastases.
These events were followed by studies in the late 1990s that demonstrated an association between levels of VEGF in the aqueous of patients, and active angiogenic ocular pathologies – including proliferative diabetic retinopathy, wet age-related macular degeneration and retinopathy of prematurity. Perhaps for these reasons, little thought was given to the possibility that VEGF might play a role in the adult that was not associated with vascular pathology.
The Many Lives of VEGF
In 2006, purely for the reason of scientific curiosity, we decided to examine VEGF levels in various tissues of adult mice. We were surprised to find that virtually all tissues had some – or many – types of cells that produced VEGF. As these were normal adult tissues, the VEGF was being expressed in the absence of any active angiogenesis. This prompted the question: What was the function of the VEGF being produced in adult tissues? We started to wonder whether there might be a role for VEGF in the adult that was unrelated to angiogenesis.
There were, in fact, already clues suggesting roles for VEGF in the adult that were distinct from angiogenesis, including two clinical examples where the actions of VEGF were being blocked. One was a pathology of pregnancy, preeclampsia, the early stages of which are characterized by hypertension and proteinuria. It had been shown that the plasma of severely preeclamptic woman contains particularly high levels of soluble FLT1 (FMS-related tyrosine kinase 1, also known as soluble VEGFR1). Soluble FLT1 contains the extracellular (that is, ligand-binding) portion of a high affinity VEGF receptor. As FLT1 circulates, it sequesters free VEGF, preventing it from binding to its receptor; in effect, neutralizing VEGF. The study showed that symptoms of preeclampsia could be replicated by administering soluble FLT1 to pregnant rats, demonstrating that VEGF neutralization played a central role in the pathogenesis.
Further evidence came from patients with cancer who received intravenous anti-VEGF antibody (bevacizumab) as a form of adjuvant chemotherapy. The circulating antibodies mopped up not only tumor-derived VEGF, but also any other available VEGF, thereby neutralizing the action of VEGF systemically. Interestingly, the primary side effects of bevacizumab were (like preeclampsia) proteinuria and hypertension. Thus, it was clear that VEGF was, indeed, playing a role in the adult that was unrelated to its ability to stimulate new blood vessel growth.
The biological basis for the proteinuria associated with systemic VEGF neutralization is now known. Our earlier work revealed that kidney podocytes (an epithelial cell that lies in very close proximity to the glomerular capillaries) express high amounts of VEGF. The glomerulus, like many filtrating organs and tissues (such as the ciliary body or the pancreas) is comprised of capillaries that are fenestrated (that is, have pore-like structures that maximize the exchange between blood and tissues). It has since been demonstrated by a number of researchers that all fenestrated capillaries are dependent on a local source of VEGF – virtually always epithelial cells – that is essential for the maintenance of both the fenestrations and the fenestrated capillary endothelium itself.
VEGF in the Normal Eye
Motivated by these clinical observations and by the knowledge that VEGF is ubiquitously expressed in adults, we set out to understand what role VEGF might play in the adult eye under normal conditions. As we had already observed VEGF production in most ocular tissues – the retina, the lens and the ciliary body (4–6) – we suspected that VEGF blockade would have effects in the normal adult eye.
The retina has many VEGF-expressing cells, including pericytes and astrocytes in the ganglion cell layer, Müller cells, and the retinal pigment epithelium (Figure 1). However, the fact that cells in the retina produce VEGF is, of itself, not evidence that VEGF has a function: we needed to know if the VEGF receptor was also expressed. Using a variety of methods, we demonstrated that a number of cells in the retina express VEGFR2, the primary signaling receptor for VEGF (Figure 1). These include the capillary endothelium of the retina, choroid and the ciliary body, Müller cells and, most surprisingly, photoreceptor cells. The concurrent presence of cellular sources and targets of VEGF strongly suggested that this factor could be involved in the maintenance of the retina’s integrity and/or its function.
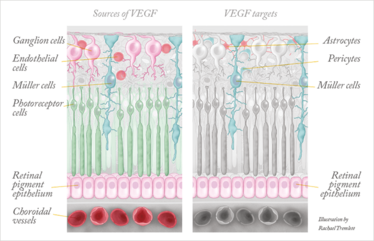
Figure 1. Cellular sources and targets of VEGF in the normal adult retina. In the adult retina and under normal conditions, VEGF is expressed by various cells types including pericytes, astrocytes, Müller cells and retinal pigment epithelium (left panel). Cellular targets of VEGF, that is cells expressing the primary signaling receptor VEGFR2, include the retinal and choroidal vessel endothelium, the ganglion cells, the Müller cells, the photoreceptors and the retinal pigment epithelium (right panel). This pattern of expression suggests that VEGF signaling may be important for the maintenance and function of the vascular, glial, neuronal and epithelial components of the adult retina.
To investigate this in an experimental system, we intravenously injected into mice adenovirus that expressed soluble FLT (the culprit in preeclampsia). Adenoviruses primarily lodge in the liver, effectively becoming a factory for the production of soluble FLT and leading to systemic VEGF neutralization, which enabled us to study the impact of VEGF neutralization on various tissues.
When we looked at the histology of the retina after two weeks of VEGF neutralization, we found that many cells in the inner and outer nuclear layer showed signs of damage and cell death. Further examination at 28 days revealed a significant thinning of the retina (Figure 2) and vision loss, indicated by a reduction of the electroretinograph a- and b-waves. These observations were consistent with the pattern of expression of VEGF and its receptor, with VEGF derived from Müller cells likely acting as a survival factor not only for Müller cells themselves, but also for the neighboring photoreceptors – VEGF appeared to signal in both an autocrine and paracrine manner. This hypothesis was supported by additional tissue culture studies, in which we demonstrated that freshly isolated photoreceptors cultured in low nutrient media die unless they are protected by the addition of VEGF.
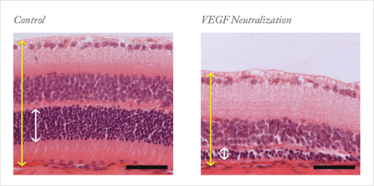
Figure 2. VEGF neutralization causes photoreceptor loss in mice. Mice expressing high level of soluble FLT1 (right) for four weeks showed significant thinning of the retina (yellow arrows), particularly of the outer nuclear layer (ONL, white arrows), compared to control mice (left). Bar is 50µm.
Using the same SFLT-1 overexpression mouse model, we went on to examine the effect of VEGF blockade on the ciliary body (6). Located in the anterior segment, the ciliary body produces the aqueous humor and mediates lens accommodation. Each ciliary process consists of centrally located fenestrated capillaries overlaid with a double layer of epithelium, an inner pigmented epithelium and an outer non-pigmented epithelium. We determined that VEGF is produced by the pigmented epithelium, whereas VEGFR2 is expressed by the fenestrated endothelium and the non-pigmented epithelium. Consistent with this expression pattern, ciliary bodies from mice with systemic VEGF neutralization suffered from degeneration of the non-pigmented epithelium and the capillaries. These changes included the appearance of vacuoles (a possible sign of autophagy), thinning of the epithelium, loss of capillary fenestrations, and the appearance of microthrombi. This degeneration was associated with impaired ciliary body function, as evidenced by a decrease in intraocular pressure. These observations parallel what is observed in the choroid plexi in the brains of mice that had been subject to VEGF blockade (7). Like the ciliary body, the choroid plexus is a secretory tissue – the site of cerebral spinal fluid production – and is comprised of a core of fenestrated capillaries covered by VEGF-producing epithelial cell layers.
We also investigated the function of the VEGF that is produced by the retinal pigment epithelium (8). Once again, we demonstrated that the retinal pigment epithelial cells, along with the endothelial cells of the underlying choriocapillaris, express VEGFR2. This pattern of expression indicated that VEGF might act in an autocrine manner on retinal pigment epithelial cells and on underyling choriocapillaris endothelial cells by paracrine signaling. To test whether the VEGF from the retinal pigment epithelium was important for maintenance of the choriocapillaris, we used a mouse model in which the form of VEGF produced by the retinal pigment epithelium could not effectively reach the choriocapillaris endothelial cells but would still be able to act on retinal pigment epithelial cells.
To understand how this was accomplished it is important to know that VEGF in the mouse is produced as three isoforms of different molecular weights (they all come from the same gene and are produced by alternative splicing). The longest isoform (referred to as VEGF188) is highly charged so cannot easily diffuse away from the pigment epithelium after it is secreted. Retinal pigment epithelial cells do not normally make any VEGF188. Instead they make the more diffusible forms, VEGF120 and VEGF164. This makes sense if VEGF needs to reach the choriocapillaris on the other side of Bruch’s membrane. We genetically engineered mice so that the pigment epithelium could make only VEGF188. These mice exhibited age-dependent degeneration of both the retinal pigment epithelium and the choriocapillaris; by the time the mice were 18 months old (equivalent to old-age in humans), degeneration of the retinal pigment epithelium and a loss of the choriocapillaris and photoreceptor cells could be observed. The changes in the retinal pigment epithelium of the choroid in the mice were not unlike those seen in human geographic atrophy.
Implications for Anti-VEGF Therapy
What do these experimental observations mean for the use of intravitreal anti-VEGF? It is important to point out that the results described above were obtained in experimental models that involve a continuous and high-level blockade of VEGF. This contrasts with the clinical situation, where the anti-VEGF antibodies injected intravitreally are cleared relatively quickly: the reported half-lives are 5.6, 3.2 and 4.8 days for bevacizumab, ranibizumab and aflibercept, respectively. In addition, anti-VEGF treatments are administered only every 4–12 weeks. Thus, in the clinical situation, VEGF neutralization may be complete in the short-term, but the antisera are cleared and the neutralization does not persist so, meaning that levels of both normal and pathologic VEGF are restored.
The restoration of physiologic VEGF in anti-VEGF-treated patients likely accounts for the fact that the undesired side effects are few (or take a long time to appear). Pathologic VEGF is similarly “reestablished” – that’s why the vessel leakiness and vision loss returns – necessitating periodic retreatment with anti-VEGF therapy.
Our findings in mouse models do, however, raise questions about chronic intravitreal anti-VEGF treatment (Figure 3). There is increasing evidence that long-term therapeutic VEGF neutralization may have undesirable side effects. The loss of photoreceptors – and vision – in patients treated with anti-VEGF agents in the absence of choroidal neovascularization (CNV) has led to speculation that the these drugs may interfere with the neuroprotective actions of VEGF, as predicted and supported by the studies described above (9). In addition, spectral domain optical coherence tomography (OCT) studies performed after six and 12 months of anti-VEGF treatment revealed “significant thinning of the choroid, whereas control eyes showed no reduction in the choroidal thickness over six months” (10). Similarly, thinning of the nerve fiber layer has been detected following anti-VEGF treatment (11). This is of particular concern for the subset of patients with AMD also affected by glaucoma for which the nerve fiber layer is already vulnerable.
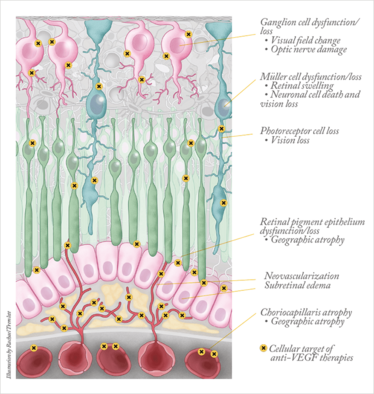
Figure 3. Cellular targets and potential side effects of anti-VEGF therapies in AMD. By blunting the neovascular process and reducing the retinal edema, anti-VEGF therapies are able to efficiently block disease progression and improve visual function of patients with wet AMD. However, anti-VEGF treatments are not specific to the pathological processes and may also neutralize physiological VEGF signaling on other retinal targets cells (ganglion cells, Müller cells, photoreceptors, retinal pigment epithelium and choriocapillaris endothelium). Long-term blockade of normal VEGF pathways may induce retinal cell dysfunction and/or death, leading to serious deleterious effects such as worsening of disease and visual loss.
There are also a number of reports documenting sustained elevations in intraocular pressure following intravitreal anti-VEGF treatment, which appear to be associated with more frequent injections. The mechanisms underlying this effect have not been elucidated; however, it is consistent with our observations of the effect of VEGF blockade on the integrity of the ciliary body.
Clearly, the availability of anti-VEGF therapy for a variety of ocular pathologies characterized by edema and/or neovascularization has been life changing, for both patients and clinicians. However, these recent observations indicate that the additional, physiological, roles of VEGF in the eye must be considered. Treatment regimes that are driven by need – that is, pro re nata (PRN) – would seem to be preferable to scheduled monthly treatments. Our data also caution against any move towards continuous release of anti-VEGF agents: total VEGF blockade would shut down physiological VEGF and is unlikely to be ideal. The goal should clearly be to target the pathological VEGF while sparing the physiological.
Magali Saint-Geniez and Patricia A. D’Amore are at the Schepens Eye Research Institute, Massachusetts Eye and Ear Department of Ophthalmology, Harvard Medical School, Boston, MA, USA.
- DW Leung, G Cachianes, WJ Kuang, et al., “Vascular endothelial growth factor is a secreted angiogenic mitogen”, Science, 246(4935), 1306–9 (1989).
- DR Senger, SJ Galli, AM Dvorak, et al., “Tumor cells secrete a vascular permeability factor that promotes accumulation of ascites fluid”, Science, 219(4587), 983–5 (1983).
- PJ Keck, SD Hauser, G Krivi, et al., “Vascular permeability factor, an endothelial cell mitogen related to PDGF”, Science, 246(4935), 1309–12 (1989).
- ASR Maharaj, M Saint-Geniez, AE Maldonado, PA D’Amore, “Vascular endothelial growth factor localization in the adult”, Am J Pathol., 168(2), 639–48 (2006).
- M Saint-Geniez, ASR Maharaj, TE Walshe et al., “Endogenous VEGF is required for visual function: Evidence for a survival role on Müller cells and photoreceptors”, PLoS One, 3;3:e3554 (2008).
- KM Ford, M Saint-Geniez, TE Walshe, PA D’Amore, “Expression and role of VEGF-A in the ciliary body”, Invest Ophthalmol Vis Sci., 53, 7520–7527 (2012).
- ASR Maharaj, TE Walshe, M Saint-Geniez, et al., “VEGF and TGF-β are required for the maintenance of the choroid plexus and ependyma”, J Exp Med., 205(2), 491–501 (2008).
- M Saint-Geniez, T Kurihara, E Sekiyama, et al., “An essential role for RPE- derived soluble VEGF in the maintenance of the choriocapillaris”, Proc Natl Acad Sci USA, 106, 18751–6 (2009).
- PJ Rosenfeld, H Shapiro, L Tuomi, et al., “Characteristics of patients losing vision after 2 years of monthly dosing in the phase III ranibizumab clinical trials”, Ophthalmology, 118, 523–530 (2011).
- L Branchini, C Regatieri, M Adhi, et al., “Effect of intravitreous anti-vascular endothelial growth factor therapy on choroidal thickness in neovascular age-related macular degeneration using spectral-domain optical coherence tomography”, JAMA Ophthalmol., 131, 693–694 (2013).
- M Martinez-de-la-Casa, A Ruiz-Calvo, F Saenz-Frances, et al., “Retinal nerve fiber layer thickness changes in patients with age-related macular degeneration treated with intravitreal ranibizumab.” Invest Ophthalmol Vis Sci., 53, 6214–621 (2012).
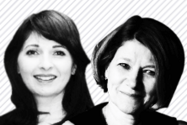
Magali is an Assistant Scientist at the Schepens Eye Research Institute, and an Assistant Professor of Ophthalmology at Harvard Medical School. Her research interests focus on the development of new strategies for the characterization and treatment of retinal degenerative diseases such as AMD. Magali also enjoys directing educational videos like “Can Treat This,” that tell the story of how to treat AMD (see http://top.txp.to/damore/amd).
Patricia holds many learned positions at Harvard Medical School, including Director of Research at the Schepens Eye Research Institute; Vice Chair of Basic Research in Ophthalmology; the Charles L. Schepens Professorship of Ophthalmology; Professor of Pathology, and Co-Director of the AMD Center of Excellence. Patricia participates in the Susan G. Komen 3-Day, 60 miles walk. To date, she’s completed eight walks, totaling 480 miles in the process, plus another 1,500 miles in training.