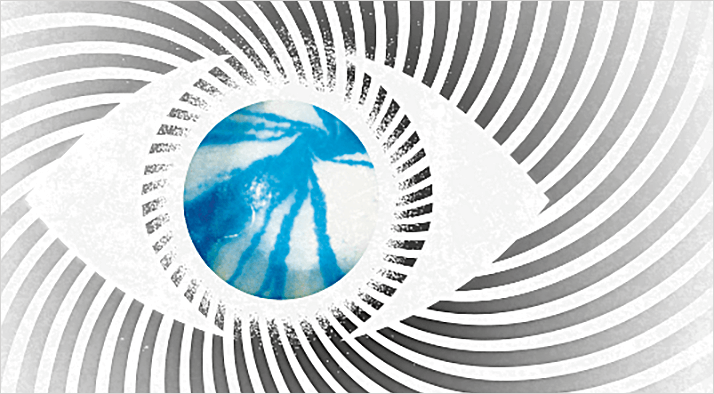
- Despite the fact that hundreds of thousands of corneal interventions are performed each year, our understanding of how the cornea is built and maintained is far from complete.
- Mouse chimera studies (where cells from one lineage can be stained blue) revealed mice with stripy corneas!
- This suggests that stem cells in the basal layer of the limbal epithelium produce cells that move radially towards the center of the cornea and continue to proliferate as they do so.
- A better understanding of corneal cell biology and development will help inform future clinical studies – particularly research involving corneal stem cells.
With hundreds of thousands of corneal interventions, including transplants, debridements and laser surgery being performed annually, you could be forgiven for thinking that we have a fairly complete understanding of how the ocular surface is maintained and how it responds to injury. It is, in fact, a highly contentious topic that today divides the scientists who work in this area. Given that the integrity of the cornea is essential for vision, resolving the current controversies in the field will impact on clinical practice and could improve the prognosis for those with severe ocular surface injury or disease. Here we describe how we serendipitously stumbled into the world of ocular surface epithelial biology, and what it has meant for our understanding of the roles of corneal stem cells.
In the beginning, there were chimeras
Our work on mouse models of corneal maintenance began in 1996. John’s research group in the University of Edinburgh was making mouse chimeras. At the time, the group’s interest was in reproductive and developmental biology, but even then, eyes were on the agenda as John was collaborating with Bob Hill of the MRC Human Genetics Unit on the other side of the city – studying the role of the Pax6 gene in mouse eye development. Martin joined the research group to strengthen this part of the work.Formally, a chimera is a composite organism containing two or more lineages of genetically distinct cells that are derived from different zygotes. It may be easier to think of it as being the opposite of identical twins: whereas one zygote splits to produce two separate identical twins, chimeras are the result of cells from two zygotes coming together to form a single embryo. One way of producing mouse chimeras experimentally is to collect 8-cell stage embryos from two different strains of mice (e.g. pigmented and albino), aggregate them in pairs (one from each strain) and culture them overnight (Figure 1b). By the next morning each pair will have amalgamated into a single chimeric embryo, and groups of these chimeric embryos can be surgically transferred to female mice to complete development. If all goes to plan, chimeric pups are born. Amazingly, these chimeras are normal sized, perfectly formed animals. It would be easy to imagine them being twice the size, or having two heads, but early mammalian embryos exhibit a property of regulation, whereby they can recover from sometimes quite significant injuries or manipulations and restore normal development. It is this property of regulation that, for example allows clinical scientists to remove one or two cells from an early human embryo for genetic testing after in vitro fertilization, without danger of harming the baby.
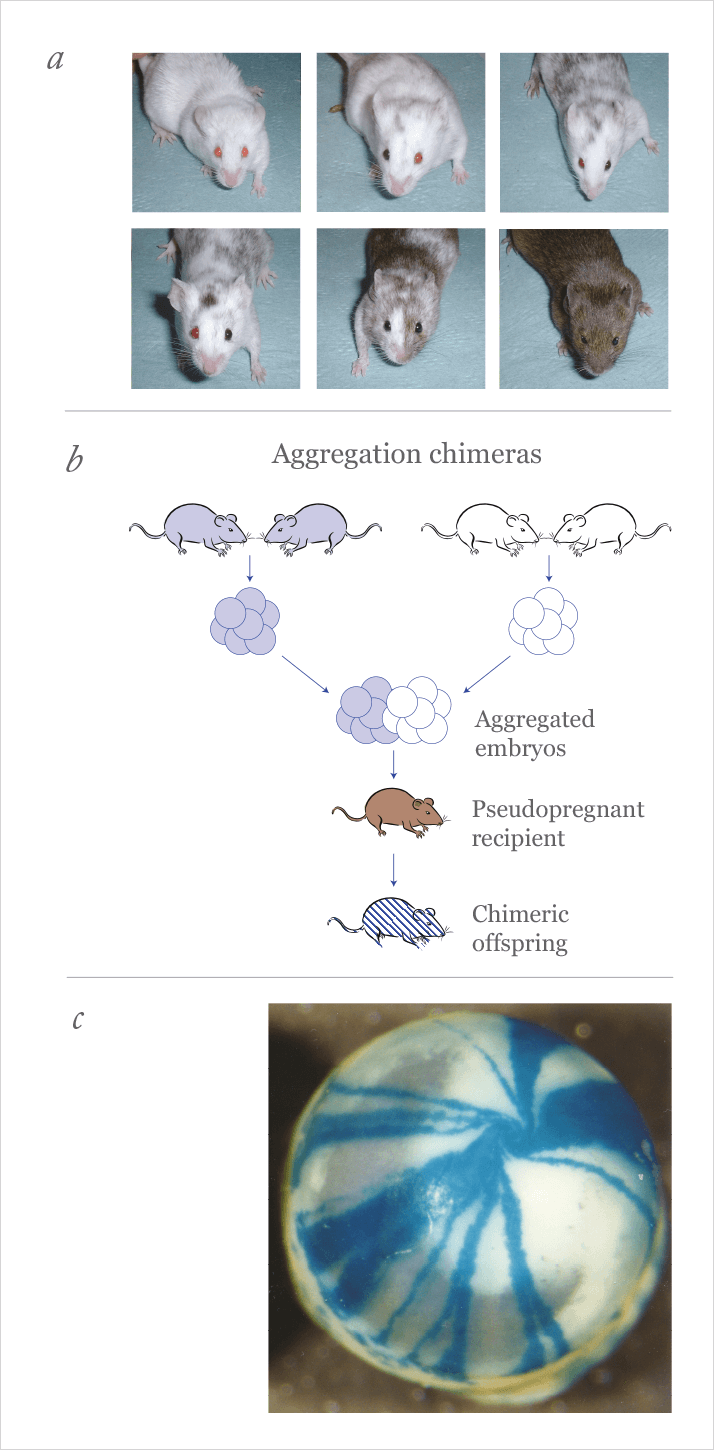
If one of the two embryos in a chimeric aggregate carries a suitable genetic marker, the distribution of the two embryonic lineages can be traced in different tissues of the chimera. The simplest type of marker is pigment. For example, if a chimera is made by aggregating an embryo from a pigmented mouse strain with one from an albino mouse strain, the distributions of pigmented and albino cells in tissues, such as the retinal pigment epithelium (RPE) and the coat, can reveal information about how these tissues grow and patterns of cell migration (Figure 1a). Transgenic markers, such β-galactosidase (β-gal) from the bacterium Escherichia coli and green fluorescent protein (GFP) from the jellyfish Aequorea victoria, have allowed the same approach to be used in non-pigmented tissues and this has proved extremely useful for many biological studies with chimeras (1).
Chimeric corneas with stripes
Back in the 1990s we were exploring the use of a β-gal marker in different tissues of fetal and adult mouse chimeras. Basically, cells from this embryo carry the LacZ transgene, and go on to express the β-gal enzyme – and these cells can be stained blue. In most tissues, the blue-stained cells formed rather confused patterns of randomly oriented patches, which reflect the chaos of randomly oriented growth and cell division during embryogenesis. It was a totally different story in the eye: the adult cornea produced beautifully clear patterns of radial stripes, often with a tight spiral at the center (Figure 1c) − rather like children’s marbles! From that moment we were hooked on the cornea – what could cause these amazing stripes? We already knew that Lawrence Bodenstein and Richard Sidman at Harvard had published elegant work explaining how pigmented and albino stripes formed in the peripheral RPE of chimeric mice. They showed that during early post-natal development, the dividing cells were mainly at the edge of the growing RPE and this edge-biased growth caused the stripes to extend outwards as the tissue enlarged (2,3). Our first thought was that the stripes in the corneal epithelium must also arise during development and extend outwards as the cornea grew. However, our preliminary work showed that this was not the case and, as we had no other ready explanation, the work was shelved for a few years.Enter the ophthalmologists
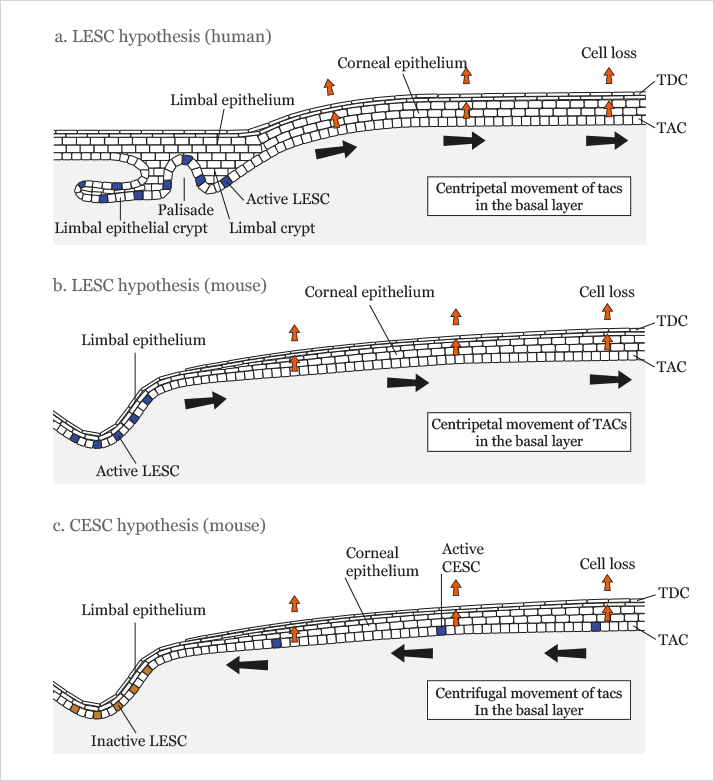
The penny dropped in 2001 when we met two Edinburgh-based ophthalmologists, Baljean Dhillon and Kanna Ramaesh, to discuss our work on Pax6 mutant mouse eyes. In conversation, they mentioned that it was widely believed that the corneal epithelium was maintained by stem cells at the periphery of the cornea in the corneal limbus. According to this limbal epithelial stem cell (LESC) hypothesis (Figure 2a,b), the stem cells that maintain the corneal epithelium are all located in the basal layer of the limbal epithelium.
They produce transient (or transit) amplifying cells (TACs), which move radially towards the center of the cornea and continue to proliferate as they go, producing the more differentiated cells in the suprabasal cell layers, which are eventually lost from the surface. The LESC hypothesis immediately suggested a new explanation for the radial stripes in the corneas of our mouse chimeras. It seemed likely that β-gal-positive stem cells in the limbus produced clones of β-gal-positive cells that moved into the cornea and continued to move across the corneal radius to the center, thereby forming radial stripes. We then set out to explore the nature of the radial striped pattern in mouse corneas in order to see if it could help us understand how stem cells manage to maintain the corneal epithelium.
Of course, it’s not as simple as it sounds. The structure of the limbus differs slightly between humans and mice. The human limbal epithelium is thicker than the corneal epithelium and has a series of folds, called palisades of Vogt. It has been suggested that crypts between, or peripheral to, the palisades may provide the required niche environment for maintaining human LESCs (4,5). In contrast, the mouse limbal epithelium is thinner than the central corneal epithelium and, like many other species, the mouse lacks the palisades and associated crypts. Nevertheless, the basic biology of corneal epithelial maintenance is likely to be similar across mammalian species and given the formidable array of genetic resources now available for studies with mice, they are indispensable models for most biomedical systems, including the cornea.
Patches are replaced by stripes after stem cells are activated
Having identified radial patterns of stripes in the corneas of adult chimeric mice (Figure 1c), we replicated the observation with a simpler experimental system using female, X-inactivation mosaic mice, which carried a LacZ marker transgene (XLacZ) on one X-chromosome (6) (Figure 3a,b). Because, in female mammals, one of the two X-chromosomes is silenced independently in every cell of the early embryo (more or less at random), these mosaic mice are composed of the usual jumble of blue and white cells we saw before with LacZ chimeras. Once again, however, the adult corneal epithelium produced a striking pattern of radial stripes. What particularly caught our attention was that before about five weeks of age, the blue-stained, β-gal-positive cells were arranged as a randomly oriented patchwork. However, this pattern changed dramatically from patches to radial stripes over a few weeks (Figure 3c), which implied that LESCs do not become active until several weeks after birth.
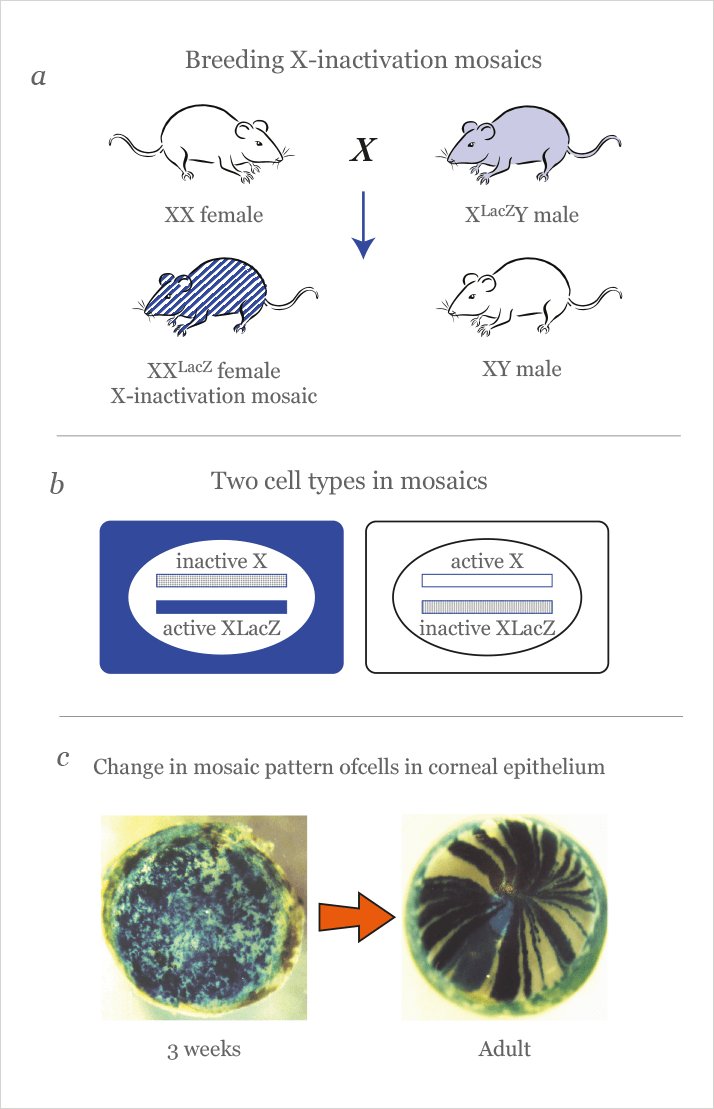
Assuming that the postnatal pattern of patchy β-gal-positive cells represents the stem cell-free random growth of the corneal epithelium, produced during development, its eventual replacement by radial stripes fitted well with the LESC hypothesis. Once activated, LESCs would produce new TACs that could move radially into the corneal epithelium, so replacing the original population of corneal epithelial cells, as they are lost by abrasion (7). Similar results have now been described in other mouse experimental systems (8–10).
The emergence of stripes at the periphery of the cornea and their extension across the corneal radius fitted nicely with the prevailing LESC hypothesis – but this narrative was soon challenged. François Majo and his team in Lausanne, Switzerland tested the LESC hypothesis surgically by transplanting β-gal-positive mouse limbal tissue to the limbus of β-gal-negative, immunocompromised mice, the transplanted limbal tissue failed to contribute to the corneal epithelium unless the host corneal epithelium was removed (13). So, although the transplanted limbal tissue contributed to corneal repair, it did not contribute to steady state corneal maintenance during normal tissue homeostasis – as was predicted by the LESC hypothesis. This surprising result prompted the authors to suggest the alternative corneal epithelial stem cell (CESC) hypothesis (Figure 2c), which proposes that the corneal epithelium is normally maintained by stem cells (CESCs) scattered throughout the corneal epithelium itself. This alternative hypothesis also recognizes the existence of LESCs but they are assumed to be active only during wound healing and so would play no role in normal maintenance of the unwounded corneal epithelium.
This new CESC hypothesis caused a storm of controversy in the field and was criticized for not accounting for several previous observations (14), including the evidence from mosaic mice that radial stripes emerge from the limbus and replace the original patchy mosaic cell distribution. On the other hand, the LESC hypothesis has difficulty explaining why cells from transplanted limbal tissue failed to colonize the host corneal epithelium. Perhaps the CESC hypothesis applies to mice but not to humans? The problem is that the LESC evidence also came from mice. So how do you solve the LESC vs. CESC dilemma? From a clinical perspective, does it even matter which hypothesis is correct?
In some respects it doesn’t. Both hypotheses agree that stem cells exist in the limbal epithelium – and it’s widely recognized that this region is an excellent source of cells for therapeutic use. Indeed limbal tissue transplantation and limbal stem cell therapy have been developed as successful treatments for visual impairment caused by conditions described collectively as limbal stem cell deficiency (15,16). However, to understand the biology of corneal epithelial maintenance, it is critical to know where the stem cells that maintain the tissue during normal homeostasis are located. If corneal epithelial cells really can show stem cell activity, at least under experimental conditions, this would offer new possibilities of therapeutic strategies for injured or diseased corneas.
Resolving the debate: next steps
Despite the controversy, François Majo’s limbal transplantation results (13) might be compatible with the LESC hypothesis if their surgical manipulation perturbed normal homeostasis and so affected the outcome. To test this, what we need is a method that can label some of the putative stem cells in the adult ocular surface without disturbing homeostasis. Sophisticated, inducible lineage tracing methods using transgenic mice are now available that can be used to throw a genetic switch to label individual stem cells present in either the limbus or cornea with a fluorescent or histochemical marker that will identify them and all their mitotic progeny. The labeled stem cells would then produce clones of labeled daughter TACs and it should be possible to identify whether they arise in the limbal epithelium and move centripetally to the cornea or if they arise in the corneal epithelium itself. Such methods have already been used to trace stem cell lineages in other tissues, including the intestinal epithelium (17). We, along with several other research groups, are beginning to use this approach to trace putative stem cell lineages in the mouse ocular surface. The expected endpoint is that transgenic mice will produce a relatively small number of individual, long-lived clones in the form of radial stripes, where each stripe emerges from a single adult stem cell. Identifying the origin of such stripes and the way they change with time after labeling is induced should help resolve the LESC vs. CESC debate in the near future. We are optimistic that the inducible stripes produced by this type of lineage-tracing approach will provide even greater insight into the maintenance of the corneal epithelium than the stripes we observed with the original chimera and mosaic systems.John West is a Reader in the Centre for Integrative Physiology and School of Clinical Sciences, University of Edinburgh, Edinburgh, UK. J. Martin Collinson is a Professor in the Institute of Medical Sciences, University of Aberdeen, Aberdeen, UK.
References
- S. Eckardt, K.J. McLaughlin, H. Willenbring, “Mouse chimeras as a system to investigate development, cell and tissue function, disease mechanisms and organ regeneration”, Cell Cycle, 10, 2091–2099 (2011). doi:10.4161/cc.10.13.16360. L. Bodenstein, R.L. Sidman, “Growth and development of the mouse retinal pigment epithelium. I. Cell and tissue morphometrics and topography of mitotic activity”, Dev. Biol., 121, 192–204 (1987). L. Bodenstein, R.L. Sidman, “Growth and development of the mouse retinal pigment epithelium. II. Cell patterning in experimental chimeras and mosaics”, Dev. Biol., 121, 205–219 (1987). H.S. Dua, V.A. Shanmuganathan, A.O. Powell-Richards, et al., “Limbal epithelial crypts: a novel anatomical structure and a putative limbal stem cell niche”, Br. J. Ophthalmol., 89, 529–532 (2005). A.J. Shortt, G.A. Secker, P.M. Munro, et al. “Characterization of the limbal epithelial stem cell niche: Novel imaging techniques permit in vivo observation and targeted biopsy of limbal epithelial stem cells”, Stem Cells, 25, 1402–1409 (2007). doi:10.1634/stemcells.2006-0580. S.-S. Tan, E.A. Williams, P.P.L. Tam, “X-chromosome inactivation occurs at different times in different tissues of the post-implantation mouse embryo”, Nat. Genet., 3, 170–174 (1993). J.M. Collinson, L. Morris, A.I. Reid, et al. “Clonal analysis of patterns of growth, stem cell activity, and cell movement during the development and maintenance of the murine corneal epithelium”, Dev. Dyn., 224, 432–440 (2002). M. Endo, P.W. Zoltick, D.C. Chung, et al., “Gene transfer to ocular stem cells by early gestational intraamniotic injection of lentiviral vector”, Mol. Therap. 15, 579–587 (2007). W. Zhang, J. Zhao, L. Chen, et al., “Abnormal epithelial homeostasis in the cornea of mice with a destrin deletion”, Mol. Vis., 14, 1929–1939 (2008). Y. Hayashi, N. Watanabe, Y. Ohashi, “The ‘Replacement Hypothesis’: Corneal stem cell origin epithelia are replaced by limbal stem cell origin epithelia in mouse cornea during maturation”, Cornea, 31, Suppl. 1, S68–S73 (2012). doi: 10.1097/ICO.0b013e318269c83f. R.L. Mort, T. Ramaesh, D.A. Kleinjan, et al., “Mosaic analysis of stem cell function and wound healing in the mouse corneal epithelium”, BMC Dev. Biol. 9, 4 (2009). doi: 10.1186/1471-213X-9-4. L.J. Leiper, J. Ou, P. Walczysko, et al. “Control of patterns of corneal innervation by Pax6”, Invest. Ophthal. Vis. Sci. 50, 1122–1128 (2009). doi: 10.1167/iovs.08-2812. F. Majo, A. Rochat, M. Nicolas, et al., “Oligopotent stem cells are distributed throughout the mammalian ocular surface”, Nature, 456, 250–255 (2008). doi: 10.1038/nature07406. T.T. Sun, S.C. Tseng, R.M. Lavker, “Location of corneal epithelial stem cells”, Nature, 463, E10, doi:10.1038/nature08805 (2010). K.R. Kenyon, S.C.G. Tseng, “Limbal autograft transplantation for ocular surface disorders”, Ophthalmology, 96, 709–722 (1989). G. Pellegrini C.E. Traverso, A.T. Franzi, et al. “Long-term restoration of damaged corneal surfaces with autologous cultivated corneal epithelium”, Lancet, 349, 990-993 (1997). doi:10.1016/s0140-6736(96)11188-0. N. Barker, J.H. van Es, J. Kuipers, et al. “Identification of stem cells in small intestine and colon by marker gene Lgr5”. Nature, 449, 1003–1007 (2007).