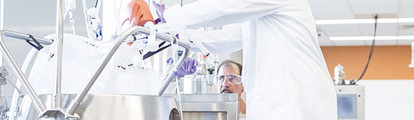
The Optogenetic Option
Optogenetics holds the potential of reversing blindness in retinal disease – but how far has the field come, and who are the key players?
At a Glance
- Optogenetics, a technique that originated in neuroscience research, allows neuronal activity to be controlled with light – and potentially bypass damaged photoreceptors to help partially restore vision in those with retinal degenerative disease
- Many of the methods under development are now poised to move to the clinical trial stage, with some prototype manufacturers working towards FDA approval for their devices
- Subretinal implants, biomimetic goggles and programmable computer chips are among the devices that may soon work with optogenetic methods to restore vision in patients with photoreceptor damage
- Photoreceptor rejuvenation or regeneration may remain the most direct path to recovering vision, but photopharmacological switches, retinal prostheses and optogenetics continue to evolve
In the battle to restore vision for those with lost or damaged photoreceptors, progress is being made on many fronts. I have previously described the possibility of reversing blindness by regenerating retinal photoreceptors (1). But there is another promising approach – optogenetics. There are a number of universities and companies currently working in this field, and in this article I will attempt to summarize the current state of research and detail how close we are to human trials of optogenetics-based techniques for restoring lost vision.
Why optogenetics?
Many blinding diseases result from the dysfunction and/or death of rod and cone photoreceptors – but in many retinal diseases, even when photoreceptor function is lost, non-photoreceptor retinal cells remain largely intact, and potentially capable of function (2).
The ganglion and bipolar cells of the retina are among those that remain active long after their attached rods and cones have atrophied. These cells are an essential part of the visual system, downstream of the photoreceptors, sending the electrical signals generated by rods and cones along the visual pathway to the brain. Because they remain relatively healthy, they represent good targets for the introduction of light-activated optogenetic proteins which could act to restore partial vision (see Figure 1).
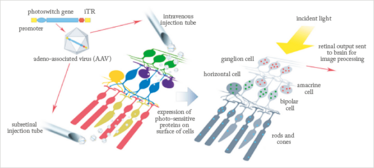
Figure 1. In optogenetics, gene therapy is used to introduce light-activated optogenetic proteins to the retinal ganglion and bipolar cells. Light signals trigger the sending of electrical signals along the optic nerve to the brain, partially restoring vision.
There are two types of chemicals that may be used to add photosensitive materials to the intact retinal cells – the light-sensitive optogenetic proteins, and the so-called photoswitches, or light-sensitive small molecules. The former are long-acting, light-sensitive channels or pumps, whereas the latter tend to be shorter-lived and require more frequent replacement. Both approaches require delivery of the photosensitive materials to the target tissue, usually either by intravitreal or subretinal injection.
The use of genetically encoded optogenetic proteins, delivered by viral carrier, may be able to offer a one-time treatment with a long-lasting effect – an exciting prospect that has already attracted a variety of researchers in both industry and academia…
Bionic Sight
Sheila Nirenberg of Weill Cornell Medical College and William Hauswirth of the University of Florida have developed a unique combination of technology that might enable those without functioning photoreceptors to “see” again. Their technology is composed of an “encoder” that delivers a train of electrical pulses to the remaining active retinal structure, and a “transducer”, which recognizes the code and transmits a similar pattern of electrical pulses to the visual cortex in the brain, via optogenetically-enhanced ganglion cells.
The encoder is a pair of glasses or goggles that includes a camera to capture the field of view – think of the high-resolution camera in your smartphone – a programmable computer chip that converts the pixels seen by the camera into a coded pattern of electrical pulses that are “readable”, or recognizable to the brain, and a miniature digital projector that transmits the light pulses to the portion of the retina containing the photoactivated dye (see Figure 2).
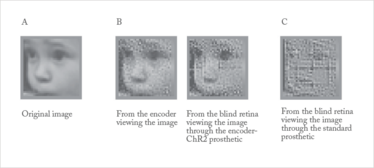
Figure 2. Images reconstructed from blind retinas fitted with the prosthetic. A. Original image. B. Left, image reconstructed from the firing patterns of the encoder. Right, image reconstructed from the firing patterns of the blind retina viewing the image through the encoder-ChR2 prosthetic. C. Image reconstructed from the firing patterns of the blind retina viewing the image through the standard (ChR2-only) prosthetic.
Work with this system took place in mice and then primates. Now that most of the basic research has been completed, the work is heavily focused on translation to patients. In the last year, the team have upgraded their prototype device to stimulate 3,000–10,000 cells in the central retina in real-time, and according to Nirenberg, they plan to meet with the FDA to map out a timeframe for human clinical trials – possibly within a year. The team have also jointly founded a company, Bionic Sight, in order to further develop their technology.
GenSight Biologics and Pixium Vision
A spin-off company from the Institut de la Vision in Paris, France, GenSight’s technology is based on R&D projects carried out in partnership with an international group of universities, as well as with the support and participation of charities including the Foundation Fighting Blindness. The company’s goal is to develop and commercialize ophthalmic therapies for the treatment of rare retinal diseases, specifically to prevent vision loss in Leber hereditary optic neuropathy and restore vision in patients with retinitis pigmentosa (RP).
GenSight’s approach combines the retinal delivery of a gene expressing a light-sensitive protein with the use of a visual interface that delivers light to activate the protein and provide visual processing functions. The product, known as GS030, is a modified AAV2 (AAV2 7m8) gene therapy vector. A DNA sequence encoding a photosensitive protein, ChrimsonR (a member of the channelrhodopsin subfamily), is introduced into the nucleus of target ganglion cells. Once in the cell, the opsins expressed by the targeted retinal cells can be stimulated by a specific wavelength of light transmitted by a set of biomimetic goggles (developed in conjunction with Pixium Vision) that enable image capture, processing and projection onto the treated retina (see Figure 3).
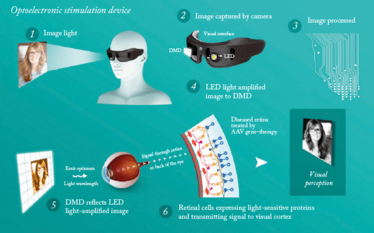
Figure 3. Vision restoration approach using GS030 and a wearable medical device. Light-sensitive proteins delivered to the retinal cells by gene therapy work with biomimetic goggles to capture, process and project an image onto the retina.
A spin-off company from the Institut de la Vision in Paris, France, GenSight’s technology is based on R&D projects carried out in partnership with an international group of universities, as well as with the support and participation of charities including the Foundation Fighting Blindness. The company’s goal is to develop and commercialize ophthalmic therapies for the treatment of rare retinal diseases, specifically to prevent vision loss in Leber hereditary optic neuropathy and restore vision in patients with retinitis pigmentosa (RP).
GenSight’s approach combines the retinal delivery of a gene expressing a light-sensitive protein with the use of a visual interface that delivers light to activate the protein and provide visual processing functions. The product, known as GS030, is a modified AAV2 (AAV2 7m8) gene therapy vector. A DNA sequence encoding a photosensitive protein, ChrimsonR (a member of the channelrhodopsin subfamily), is introduced into the nucleus of target ganglion cells. Once in the cell, the opsins expressed by the targeted retinal cells can be stimulated by a specific wavelength of light transmitted by a set of biomimetic goggles (developed in conjunction with Pixium Vision) that enable image capture, processing and projection onto the treated retina (see Figure 3).
The company is currently planning a Phase I/II, open-label, single-center trial to evaluate the safety and tolerability of GS030 and the wearable medical device. The trial will include secondary endpoints that should serve to demonstrate proof-of-concept that this approach could work in RP patients, such as the ability of GS030 treatment, with the use of biomimetic goggles, to restore a sense of vision, that is, the ability to react to visual stimuli.
LambdaVision
A startup company launched by the University of Connecticut, LambdaVision has developed a flexible, protein-based, retinal implant. The ~60 μm thick device harnesses the photochemical properties of bacteriorhodopsin, a light-activated proton pump, to create an ion gradient that stimulates the bipolar and ganglion cells within the damaged retina.
The implant is designed to be placed in the subretinal space, between the retinal pigment epithelium layer and the remaining neurosensory retinal layer (where photoreceptors are severely damaged or lost due to late-stage degeneration) (Figure 4). The implant consists of multiple layers of oriented bacteriorhodopsin on an ion-permeable substrate. Once the thin film is in place, the prosthesis absorbs incident light and initiates a unidirectional ion gradient directed toward the bipolar and ganglion cells. Vision is then generated through the indirect manipulation or competition of ions amongst native signal carriers.
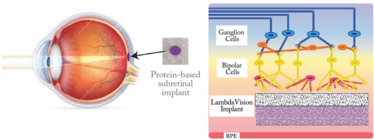
Figure 4. The LambdaVision implant absorbs incident light, and initiates an ion gradient directed toward the bipolar and ganglion cells within the retina.
RetroSense Therapeutics
RetroSense Therapeutics employ a gene therapy approach, using an AAV vector to deliver a new photosensitivity gene to retinal cells. As RP is caused by over 100 different gene defects, addressing each one individually is not feasible with current technology. RetroSense’s approach is designed to “install” modified photosensitive cells into the damaged retina, restoring vision irrespective of which gene defect is responsible for its loss.
Their current lead candidate for creating new photosensitive areas in retinal cells is RST-001, based on the photosensitivity gene channelrhodopsin-2 (CHOP2). CHOP2 is supported by a strong body of published literature; numerous studies have demonstrated its ability to restore light perception and vision in animal models of blindness, and in primate studies, its administration was well-tolerated.
The FDA has approved an Investigational New Drug Application to begin human testing of RST-001, and a clinical trial is expected to begin by the end of this year. RST-001 will be developed initially for RP, with advanced dry AMD as a follow-on indication.
A promising future?
In addition to the techniques discussed here, there are several other laboratories working on photopharmacological and even optoelectronic methods (see Table 1). It’s clear that optogenetics is a fast-moving field, with several programs already moving toward human trials. In terms of the bigger picture, it’s my belief that the rejuvenation or regeneration of photoreceptors is probably the method that should generate the best possible vision – assuming that the image processing mediated by retinal interneurons can be recapitulated with these approaches. Nevertheless, optogenetics, along with retinal prostheses, appear to be highly promising at restoring some visual function in diseases affecting the photoreceptors.
Company/ Institution and Researchers | Treatment Modality | Optogenetic Agent | Target Cells | Comment |
Friedrich Miescher Institute, Basel, Switzerland. Botond Roska | Gene therapy | Channelrhodopsin and halorhodopsin variants | Cones and bipolar cells | |
Institute for Physiology, University of Bern, Switzerland. Sonja Kleinlogel and Michiel van Wyck | Photopharmacological switches delivered via gene therapy | Chimeric receptor Opto-mGluR6 (mGluR6 basal receptor with melanopsin light antenna) | Bipolar cells | |
Newcastle University, UK. Patrick Degenaar | Micro-LED optoelectronics and gene therapy | Channelrhodopsin | Ganglion cells | |
Okayama University, Japan. Toshihiko Matsuo and Tetsuya Uchida | Photoelectric dye-coupled retinal prosthesis | OURePTM – a photoelectric dye applied to a soft thin polyethylene film | Subretinal implants | |
University of Genova, Italy. Fabio Benfenati | Optoelectronic polymer prosthesis | A flexible substrate coated by a thin layer of the photoactive polymer semiconductor | Subretinal implants | |
UC/Berkeley/Helen Wills Neuroscience Institute, US. John Flannery | Gene therapy and photopharmacological switches | Rhodopsin-enhanced gene therapy and LiGLuR and MAG0460 photoswitches | Bipolar and ganglion cells | Working on intravitreal gene therapy delivery vectors |
UC/Berkeley/Photoswitch BioSciences. Richard Kramer | Photopharmacological switches | AAQs – DENAQ & BENAQ | Ganglion cells | Working on polymer-encapsulated drug delivery system for long-term delivery |
Institute de la Vision, France. José Sahel, Deniz Dalkara, Serge Picaud, Ryad Benosman and Christoph Posch | Combined input goggles and gene therapy | Channelrhodopsin and halorhodopsin and variants | Cones, bipolar cells, ganglion cells |
Irv Arons reports on new drugs and devices for the treatment of retinal diseases. A former consultant to the ophthalmic and medical laser industries with management consultants Arthur D. Little for 25 years, Irv ran his own company, Spectrum Consulting, for 11 years before his retirement.
- I Arons, “Regenerating the Retina”, The Ophthalmologist, 5, 40–43 (2015). theophthalmologist.com/issues/0215/regenerating-the-retina/.
- B Roska, et al., “Chapter 2: Restoring Vision to the Blind: Optogenetics, The Lasker/IRRF Initiative for Innovation in Vision Science, Translational Vision Science & Technology”, Transl Vis Sci Technol, 3, [ePub only], (2014). PMID: 25653888.
Irv Arons’ Journal is a well-read blog that reports on new drugs and devices for the treatment of retinal diseases, including age-related macular degeneration (AMD). Until his retirement, Irv was a consultant to the ophthalmic and medical laser industries, working for management consultants Arthur D. Little for 25 years and running his own company, Spectrum Consulting, for 11 years.