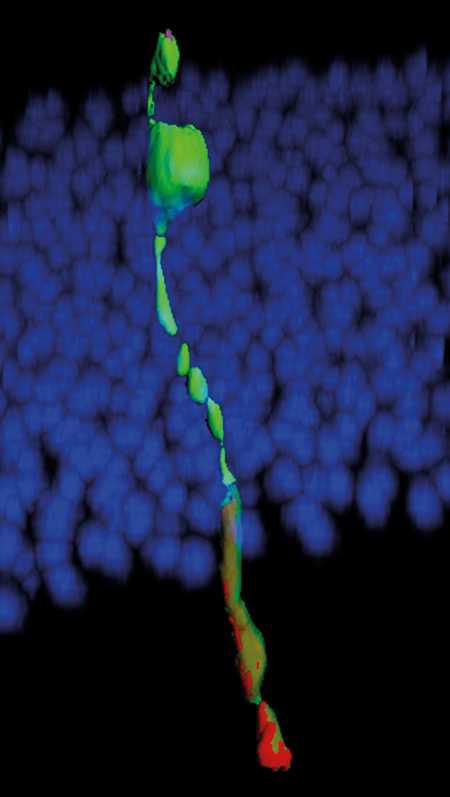
Retinal progenitor cell transplantation offers a new approach to repairing damaged photoreceptors By Irv Arons
- Retinal progenitor cells (RPCs) can replicate many times and mature into many of the major retinal cell types
- Clinical trials of RPCs for the repair of degenerated retinas are already underway
- The results so far are promising: most patients exhibit improvement in their vision, with no safety issues reported to date
- Other technologies – stem cell and gene therapy, electronic retinal implants and “retinal rejuvenation” – are also being investigated
Retinal degenerative diseases (RDDs) are a significant source of visual disability across the globe, with age-related macular degeneration (AMD) being the most common. A great number of RDDs have a genetic origin – like choroideremia, retinitis pigmentosa (RP), Leber congenital amaurosis and Stargardt disease, but irrespective of the cause, the treatment options available today are pretty limited. Wet AMD might be treatable for a period with intravitreal VEGF inhibitors, but for many patients, this only postpones the inevitable. The loss of photoreceptor cells – as seen in the later stages of RP, dry AMD geographic atrophy, and the late stages of Stargardt disease – results in permanent visual loss. There’s no approved therapy available yet that can slow or reverse the pathology. One potential way of doing this – placing retinal progenitor cells (RPCs) in the retina to replace the damaged cell types – is a therapeutic avenue that’s generating a lot of attention.
RPCs aren’t quite the same thing as stem cells. The latter can reproduce highlight, and can differentiate to become any cell type in the body. Progenitor cells are a little further along the differentiation pathway: they are still highly proliferative (though not indefinitely, like stem cells), but can only produce a subset of the cell types a stem cell can. RPCs – with the right signals – can produce each of the seven major retinal cell types (Figure 1). In other words, they’re multipotent, whereas stem cells are pluripotent… and they’ve shown great promise as a source of replacement cells in experimental models of retinal degeneration (1,2). How are retinal progenitor cells being used? With major public grants being awarded and private companies conducting their own research, RPCs are proving to be of interest to both academic and commercial investigators. Robin Ali and his group at University College London, UK, have used mouse embryonic stem cells (ESCs), differentiated to become photoreceptor precursors, and then transplanted them into adult mice with degenerated retinas (1). Not only did those cells integrate into the retinas and mature into outer segment-bearing photoreceptors, but a behavioral test, which required the mice to follow visual cues in a dimly-lit water maze, verified that vision truly was restored. Another research group, led by Henry Klassen (Associate Professor of the Gavin Herbert Eye Institute and its Stem Cell Research Center at the University of California, Irvine, Irvine [UCI], USA), opted to conduct their research in a large animal model: pigs. They obtained obtaining porcine RPCs and transplanted them into pigs with retinal dystrophy. Histologically, it looked like the RPCs were doing their intended job: first, they began expressing photoreceptor markers (suggesting appropriate differentiation),
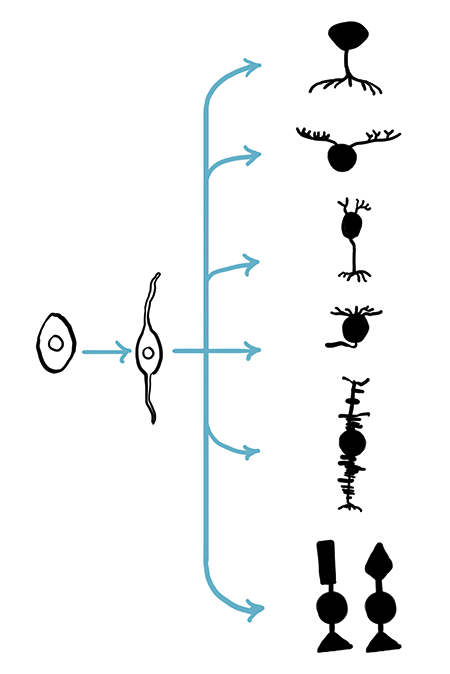
then they developed cellular polarity, and eventually self-assembled into the rudiments of outer retinal tissue (2). It’s not all pre-clinical work in animals. RPCs derived from human cells are also under investigation. A group from Central South University, China, obtained RPCs from fetal neural retinae, expanded them in vitro, and transplanted them into a rat model of retinal degeneration; the transplants not only stopped the rats’ visual decline, but rescued normal retinal morphology as well (3). Fetal retinas aren’t required for therapy – once it was established that human ESCs could be efficiently differentiated into RPCs (4), Klassen’s group developed RPCs that have already successfully been shown to restore vision after transplantation into the retinas of blind rats (5). As well as continuing this work, the group are manufacturing the cells to meet pharmaceutical standards, examining the gene expression profiles of their RPCs, and planning to commence clinical trials for the treatment of RP. Magdalene Seiler’s at UCI have conducted an early-phase clinical trial of RPCs in patients with RP (n=6) and dry AMD (n=4), who had sheets of RPCs obtained from human fetal eyes implanted into their retina. The results were promising: seven of the 10 patients exhibited improved visual acuity, and no tissue rejection was observed in any of the study participants at the 6-year follow-up visit (6). Along with Hans Keirstead (Professor of Anatomy and Neurobiology at UCI), Seiler is now developing three-dimensional sheets of human ESC-derived retinal tissue, including both progenitor and pigment epithelial cells (7), as an enhanced method of treating RDDs.
Commercial groups are also getting in on the act. Keirstead created a company, California Stem Cell, Inc. (CSC), to commercialize their work. CSC was recently acquired by the New York-based biotech company, NeoStem, and Keirstead now serves as as president of their Oncology Division. Seiler’s work continues at UCI under a grant from NeoStem Oncology. Ocata Therapeutics (formerly Advanced Cell Technology) is running three clinical trials, using human ESC-derived retinal pigment epithelium (RPE) cells for the treatment of dry AMD (8), Stargardt disease and myopic macular degeneration. Most patients report improved vision – including one whose vision went from 20/400 to 20/40 in a matter of weeks – and no safety issues have yet been recorded (9); to date, more than 30 patients have received their RPE therapy. Furthermore, the company is also pursuing a research program using photoreceptor progenitors. When injected into animals subretinally, the cells incorporated into the retina, and within a few weeks were seen moving into the outer nucleated layer and integrating into the photoreceptors, prompting regeneration of both rods and cones, and even appearing to improve retinal function (Figure 2). Klassen’s company, jCyte, is also working on bringing his own methods to clinical trials: fetal-derived RPCs, partially developed into retinal cells, for the activation of degenerating host photoreceptors and the replacement of cells lost to disease. An ambitious treatment comes from ReNeuron, a company that hopes to replace the damaged photoreceptors in patients with RP with partially developed retinal progenitors grown on a biodegradable scaffold – ReN003. The hope is that the scaffold increases the chances of the RPCs surviving, differentiating, and integrating into the host retina. Their offering is apparently close to completing its preclinical development phase, ahead of commencing a phase I/II trial in the US.
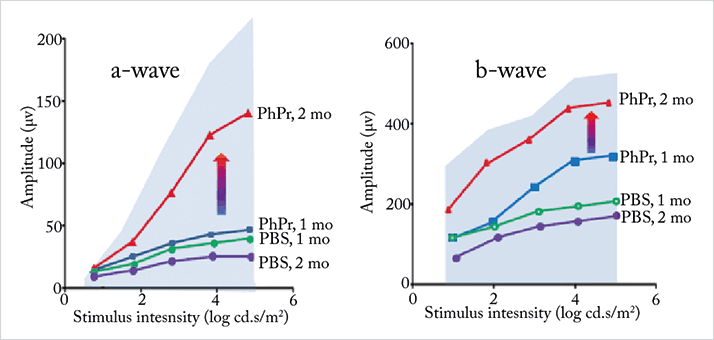
Promising alternatives Though promising, RPCs aren’t the only new technology in development. There are numerous efforts underway to restore vision to patients who have lost their sight to damaged or destroyed photoreceptors. Table 1 lists some of the most prominent alternative options. It’s incredible to think just how far we’ve come in the last 20 years – there are now five major avenues of research that are maturing quickly, with all of them holding the promise of restoring vision to the blind and the visually impaired – some are in late preclinical phases, some in early phase clinical development, and, as is the case with retinal prostheses, some are already commercially available. Many therapies fail to make it through the clinical evaluation process. There are worries about the long-term safety of all of these treatment modalities. But what data are out there – however little – looks promising; safety issues haven’t reared their ugly head so far. RPCs might (theoretically) be a little safer than stem cell or gene therapy, as no viral vectors (which risk mutagenesis) are used and the cell lines are committed to producing retinal cell types – stem cells are not. Clinical trials are commencing, and if they demonstrate that RPC implantation restores vision in people who have lost it due to damaged or destroyed photoreceptors, it is my opinion that RPC treatment will become one of the greatest advances yet seen in the battle against blindness.
Irv Arons reports on new drugs and devices for the treatment of retinal diseases. A former consultant to the ophthalmic and medical laser industries with management consultants Arthur D. Little for 25 years, Irv ran his own company, Spectrum Consulting, for 11 years before his retirement.
References
- A Gonzalez-Cordero, et al., “Photoreceptor precursors derived from three-dimensional embryonic stem cell cultures integrate and mature within adult degenerate retina”, Nature Biotechnol, 31, 741–747 (2013) PMID:23873086.
- H Klassen, et al., “Photoreceptor differentiation following transplantation of allogeneic retinal progenitor cells to the dystrophic rhodopsin pro347leu transgenic pig”, Stem Cells Int, 939801 (2012). PMID:22567027.
- J Luo, et al., “Human retinal progenitor cell transplantation preserves vision”, J Biol Chem, 289, 6362–6371. PMID:24407289.
- DA Lamba, et al., “Efficient generation of retinal progenitor cells from human embryonic stem cells”, Proc Natl Acad Sci USA, 103, 12769–12774 (2006). PMID:16908856.
- Human retinal progenitor cells as candidate therapy for retinitis pigmentosa, available here.
- ND Radtke, et al., “Vision improvement in retinal degeneration patients by implantation of retina together with retinal pigment epithelium”, Am J Ophthalmol, 146, 172–182 (2008). PMID:18547537.
- G Nistor, et al. “Three-dimensional early retinal progenitor 3D tissue constructs derived from human embryonic stem cells”, J Neurosci Methods, 190, 63–70 (2010). PMID:20447416.
- Restoring vision by sheet transplants of retinal progenitors and retinal pigment epithelium (RPE) derived from human embryonic stem cells (hESCs).
- SD Schwartz, “Human embryonic stem cell-derived retinal pigment epithelium in patients with age-related macular degeneration and Stargardt’s macular dystrophy: follow-up of two open-label phase 1/2 studies”, Lancet, Epub ahead of print (2014). PMID:25458728.
- AI Jobling, et al., “Nanosecond laser therapy reverses pathologic and molecular changes in age-related macular degeneration without retinal damage”, FASEB J, Epub ahead of print (2014). PMID:25392267.