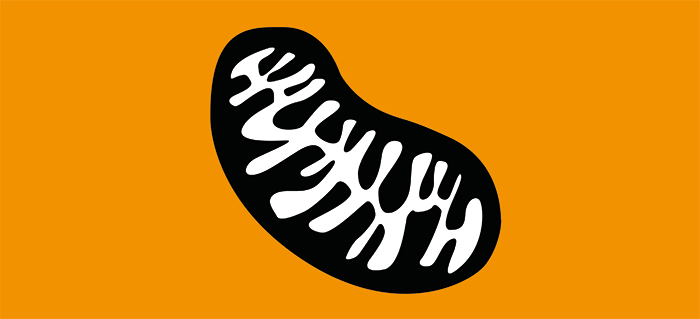
- Understanding of retinal metabolic activities and retinal melanin decay are limited, primarily because the technology has not existed to measure and study these parameters
- We set out to improve understanding by developing new imaging modalities
- Specifically, we are working with photoacoustic imaging and vis-OCT
- These new understandings will likely inform how we diagnose, stage and treat retinal disease.
Back in 2009, we were given a challenge – to optically measure the oxygen rate of the retina. It was a problem – at the time, gross retinal blood flow could be measured using laser Doppler velocimetry (and more recently, and with greater granularity, using OCT angiography). Retinal blood oxygenation saturation (sO2) can be determined using live-time fluorescence imaging or camera-based oximetry – and this approach has shown that blood flow and sO2 is altered in several pathologies, such as diabetic retinopathy and glaucoma. But there are limitations to our current knowledge regarding the retinal metabolic rate in health and disease. Why? Mainly because there is no technology that can measure both blood flow and oxygenation at the same time. To gain a more comprehensive profile of biological function and disease status, we set out to find a way to measure both.
Combining optics
Our first thought was to combine OCT with photoacoustic microscopy in the same device. As OCT angiography allows the precise determination of retinal blood flow, and photoacoustic imaging has been shown to quantify sO2 in several tissues (1), we believed that the approach would allow us to quantify everything from a single scan. I am sure there is no need to explain how OCT works to an audience of ophthalmologists, but I will offer a little more insight on photoacoustic microscopy. Principally, it is a hybrid imaging technique that detects laser-induced ultrasound (see Box – Photoacoustic Imaging Explained [1]). To make the technique suitable for retinal imaging, photoacoustic microscopy had to be modified, resulting in photoacoustic ophthalmoscopy (PAOM), an optical scanning photoacoustic microscopy system that can image retinal blood vessels and retinal pigment epithelium (RPE; 2). In 2014, we used an integrated photoacoustic ophthalmoscopy and SD-OCT system, and demonstrated that we could non-invasively measure both retinal blood flow and sO2, and quantify the retinal metabolic rate (rMRO2), in rats (3)(4).Photoacoustic imaging relies on optical absorption contrast. For biomedical imaging, optical absorption can be closely associated with physiological properties such as oxygen saturation, molecules such as hemoglobin or melanin, or from exogenous contrast agents. In photoacoustic imaging, a short-pulsed laser beam is directed into tissues; this laser energy becomes absorbed, leading to thermoelastic expansion and emission of ultrasonic – or photoacoustic – waves. An ultrasound detector detects the waves, the amplitude of which is proportional to the concentration of local optical absorbers. Axial resolution is determined by the bandwidth of the ultrasonic detector. The photoacoustic waves are then analyzed to produce reconstructed anatomical images.
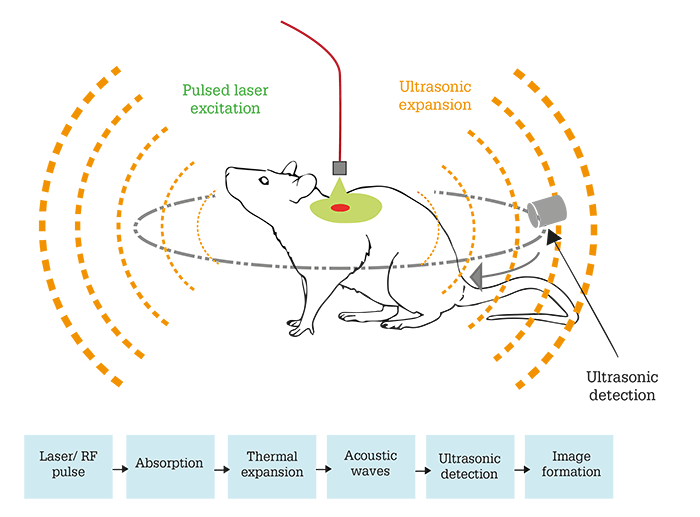
A better detector
Unfortunately, there is a major challenge with photoacoustic imaging: detection. Photoacoustic imaging was first developed for skin cancer; later on, people pushed for it to be used in brain imaging and endoscopy, but it had never been successfully used in eye imaging, primarily because a high quality detector did not exist. And we faced the same challenge: ultrasonic detection needs contact with tissue (or a coupling agent). In the early stages of our work, we used a very tiny piezoelectric transducer attached at the side of the eyelid. But everything had to be aligned too perfectly, even in mice and rats, so we realized that it was not the best way to image humans. Furthermore, many commonly used piezoelectric detectors are constrained in terms of axial resolution. To solve the ultrasonic challenge, I worked with Cheng Sun here at Northwestern University and we developed a better detector for photoacoustic imaging: the microring resonator (5). The microring resonator is a soft (or compressible) waveguide, nanofabricated on a transparent substrate, i.e. a piece of quartz (Figure 1). The waveguide has a square shape and forms a circle. It is tiny at only 60 µm in diameter and 1 µm in height, yet it is so sensitive it can detect ultrasonic pressure as low as 10 Pa, which is more than 10 times more sensitive than state-of-the-art piezoelectric detectors. It also offers an enlarged detection bandwidth that improves the axial resolution of photoacoustic microscopy (6). The combination of its small size and optical transparency means that we can detect ultrasound reflected back from the retina at a higher resolution than existing methods. For us, it seems a natural step to insert the detector into a contact lens, so that it is in contact with the eye, and we want to make it customizable so that the microring will be inserted while the contact lens is being 3D-printed. Our projection for the future is that the combined system will be used in humans; the contact lens containing the microring resonator will be placed on the patient’s eye, and together with OCT, retinal biological activities can be measured. As we are working on developing better and cheaper microring detectors, we have discovered that we’re not just limited to ophthalmic fabrications. Replacing the coverslip in traditional microscopic studies with a microring resonator-containing piece of glass means that scanning laser microscopes can become photoacoustic microscopes. This means that researchers wouldn’t have to buy a system from scratch, but can rather modify their existing system with minimal effort. We’ve also been approached by investigators to develop endoscopes, so esophagus or colon can be viewed using optical transparent detectors combined with their existing optical imaging setups. We were also recently approached by a geologist who wanted to measure seismic waves at the core of the earth!From metabolism to melanin
Measuring retinal metabolic rate might have been the major reason to begin our studies, but our other aim was to quantify melanin RPE concentration. Why? Melanin is one of the key pigments comprising the RPE, where it helps protect against photodamage and oxidative stress. But melanin concentration in the RPE decreases with aging, leaving the eye more susceptible to damage and degeneration (7)(8). Reduced concentrations of melanin in the RPE are considered to be a key indicator of the presence of age-related macular degeneration (AMD), especially in the later stages of disease, and many researchers now believe that if melanin dysfunction could be measured in the early stages of disease it could represent an early risk factor of the presence of disease. Accurately measuring RPE melanin concentration would give us more insights into both the natural history of how melanin concentration changes with age, and how this is changed as retinal diseases, such as AMD, progress. Right now, fundus autofluorescence imaging is used to measure retinal melanin concentration, typically with infrared or blue light sources. The main downside of these fundus-based imaging techniques is that they have poor axial resolution, and rely on diffused reflective photons from the retina to accumulate all the information from multiple layers, from the ganglion to photoreceptors to the RPE, and even choroid. They all mix together, and although it is not impossible to dissect information from this mixed signal, it is highly challenging. But if we could have a technology that can provide an axial resolution, say at the level of 10 µm, then we wouldn’t need to perform all these dissections, and we could image multiple layers separately. Photoacoustics is the perfect way to provide this axial resolution, and using our microring resonator on an inverted microscope system, we have for the first time, quantified the melanin concentration in both the RPE and the choroid at an axial resolution of 15 µm in samples of RPE-choroid complexes from human and porcine eyes (9)(10). In the future, we would like to explore how to quantify the melanin concentration at different stages of AMD. To do this, we are trying to monitor the melanin decay time course at different stages in a transgenic mouse model of geographic atrophy.A better way
Although photoacoustics is very sensitive, the downside is the requirement for a contact measurement. So we have developed a better technology for metabolic rate imaging – visible OCT or vis-OCT – which we now use instead of photoacoustic imaging. How does it work? Vis-OCT is much the same as traditional OCT – the key difference is that it uses light across the visible spectrum (center wavelengths: 570 nm). Most commercial OCT systems use near-infrared light (center wavelengths: 830 nm or 1,330 nm) because it is more comfortable and “patient friendly” than visible light. But to measure both blood flow and oxygenation, we had to shift the wavelength from infrared to visible light; sO2 can only be measured by OCT when in the visible spectrum (11). We’ve previously shown in rat eyes that with a single vis-OCT scan, we can obtain high quality retinal anatomical imaging (close to 2 μm; Figure 2), as well as accurate measurements of rMRO2 (12). Back in 2015, we demonstrated that vis-OCT was safe for human imaging (13) and here at Northwestern, we have now imaged over 100 patients with a range of diseases (including diabetic retinopathy, drusen formation, wet AMD and vein occlusion) referred to us by physicians. We’ve recently published results demonstrating that vis-OCT could be used to quantify sO2 in humans: in four healthy volunteers, we observed a 20 percent difference in sO2 between retinal arteries and veins (14). We’ve also used vis-OCT to show that inner retinal oxygen metabolic rate is increased in diabetic mice (15).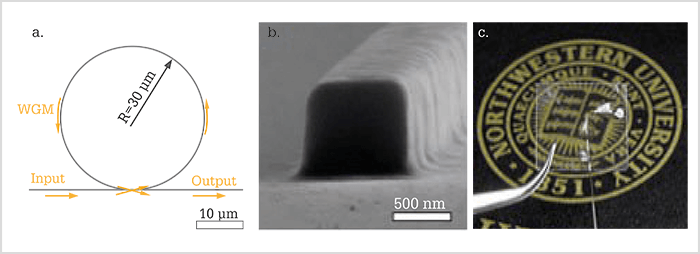
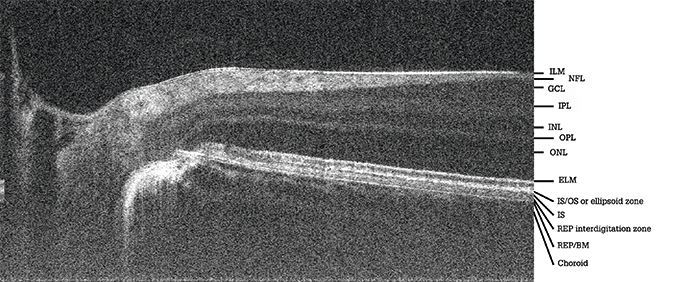
Of course, the downside of vis-OCT is that the use of visible light makes it more uncomfortable for the patient, and because visible light is blocked by the RPE, we cannot image the anatomical structures beyond this, such as the choroid, so choroidal circulation can’t be assessed. Furthermore, as the models used to calculate sO2 are based on continuous blood flow, they cannot quantify sO2 in capillaries correctly (16). Despite these limitations, the benefits with vis-OCT are a much higher axial resolution compared with any state of the art OCT, as well as a complete profiling of the metabolic signatures at the back of the eye using a single scan.
Looking ahead
In terms of photoacoustics, we want to better understand melanin decay before we begin studying it in humans. Melanin decay has not been studied historically – likely because of a lack of technology. We’re hoping to establish an approach using photoacoustic imaging based on microarray to provide an extremely sensitive way to measure melanin, and we’re currently at the laboratory validation stage. With regards to vis-OCT, we want to expand our clinical sites to collect enough patient data to submit an application to the FDA. We’re currently working with leading ophthalmologists from Northwestern University Memorial Hospital and the New York University Medical Center, but we’re also looking for more collaborators! We’d also like to develop metabolic profiles of patients with different stages of disease. It’s fair to say that we’re at the beginning of a long but exciting journey. Hao F. Zhang is Professor of Biomedical Engineering at the McCormick School of Engineering and Professor of Ophthalmology at the Feinberg School of Medicine, Northwestern University, Evanston, Illinois, USA. Disclosures: Zhang reports that he is co-founder and shareholder of Opticent Health.References
- W Liu and HF Zhang. “Photoacoustic imaging of the eye: a mini review”, Photoacoustics, 4, 112–123 (2016). PMID: 27761410. S Jiao et al., “Photoacoustic ophthalmoscopy for in vivo imaging”, Opt Express, 18, 3967–3972 (2010). PMID: 20389409. W Song et al., “A combined method to quantify the retinal metabolic rate of oxygen using photoacoustic ophthalmoscopy and optical coherence tomography”, Sci Rep, 4, 6525 (2015). PMID: 25283870. W Liu and HF Zhang. “Noninvasive in vivo imaging of oxygen metabolic rate in the retina”, Conf proc IEEE Eng Med Biol Soc, 3865–3568 (2014). PMID: 25570835. H Li et al., “A transparent broadband ultrasonic detector based on an optical micro-ring resonator for photoacoustic microscopy”, Sci Rep, 4, 4496 (2014). PMID: 24675547. B Dong, C Sun and HF Zhang. “Optical detection of ultrasound in photoacoustic imaging”, IEEE Trans Biomed Eng, 64, 4–15 (2017). PMID: 27608445. DN Su, JD Simon and T Sarna. “Role of ocular melanin in ophthalmic physiology and pathology”, PhotochemPhotobiol, 84, 639–644 (2008). PMID: 18346089. T Sarna at el., “Loss of melanin from human RPE with aging: possible role of melanin photooxidation”, Exp Eye Res, 7, 89–98 (2003). PMID: 12589778. X Shu, W Liu and HF Zhang. “Monte Carlo investigation on quantifying the retinal pigment epithelium melanin concentration by photoacoustic ophthalmoscopy”, J Biomed Opt, 20, 106005 (2015). PMID: 26469564. X Shu et al., “Quantifying melanin concentration in retinal pigment epithelium using broadband photoacoustic microscopy”, Biomed Opt Express, 8, 2851–2865 (2017). PMID: 28663911. S Chen et al., “Monte Carlo investigation of optical coherence tomography retinal oximetry”, IEEE Trans Biomed Eng, 62, 2308–2315 (2015). PMID: 25955984. J Yi et al., “Visible light optical coherence tomography measures retinal oxygen metabolic response to systemic oxygenation”, Light Sci Appl, 4, e334 (2015). PMID: 26658555. J Yi et al., “Human retinal imaging using visible-light optical coherence tomography guided by scanning laser ophthalmoscopy”, Biomed Opt Express, 6, 3701–3713 (2015). PMID: 26504622. S Chen et al., “Retinal oximetry in humans using visible-light optical coherence tomography [Invited]”, Biomed Opt Express, 8, 1415–1429 (2017). PMID: 28663838. W Liu et al., “Increased retinal oxygen metabolism preceded microvascular alterations in type 1 diabetic mice”, Invest Ophthalmic Vis Sci, 58, 981–989 (2017). PMID: 28535269. RA Linsenmeier and HF Zhang. “Retinal oxygen: from animals to humans”, Prog Retin Eye Res, 58, 115–151 (2017). PMID: 28109737. HF Zhang et al., “Visible-light optical coherence tomography oximetry in healthy individuals”, Invest Ophthalmol Vis Sci, 58, 3100 (2017).