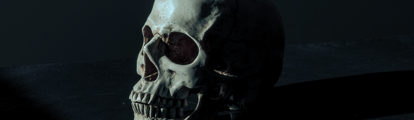
It’s in Their Bones
Bone marrow stem cells for the treatment of retinal disease? They’re closer to the clinic than you might think
At a Glance
- Adult bone marrow stem cells – including mesenchymal stem cells (MSCs) and CD34+/hematopoietic stem cells (HSCs) – may have beneficial paracrine trophic effects on the ischemic or degenerating retina
- Bone marrow MSCs are easily harvested and expanded in culture and allogeneic transplantation may be possible – but there are safety concerns when administered by intravitreal injection
- CD34+/HSCs home into the retina after intravitreal injection and may have regenerative effects in ischemic or degenerating retina
- Early clinical studies show autologous intravitreal administration of CD34+ cells from human bone marrow is possible in eyes with retinal disorders without major safety issues
We are in the middle of a demographic time-bomb. Post-war baby-boomers are now getting to the age where they’re beginning to experience vision loss from age-related eye diseases: principally cataract and retinal disorders like age-related macular degeneration (AMD), diabetic retinopathy and retinal vein occlusions. Cataract is relatively easily resolved. Retinal diseases, on the other hand, aren’t. Our current approach to the treatment of many retinal diseases is limited – and most retinal diseases are not only age-related but also result in irreversible vision loss.

But we are lucky in some respects. Retinal diseases like neovascular AMD, diabetic macular edema (DME), and the sequelae secondary to retinal vein occlusions are treatable with anti-VEGF agents, steroids, or laser therapy – unfortunately, these are not permanent solutions and disease progresses. Even if the drug treatment regimen is adhered to completely (which is essential), these agents can become less effective over time. Moreover, some vision loss is not recovered with any of the available treatments. In other words, there’s a clear unmet need for an intervention that could limit – or even better reverse – the vision loss that’s associated with these extremely (and increasingly) common retinal disorders.
Cell therapy to the rescue?
Might stem cell therapy be the answer? In theory, it has many advantages over current treatment approaches. Cell-based therapy should be able to influence more pathways and induce a broader and more physiologic effect in target tissues than conventional pharmacological interventions. They might differentiate into the cells of the target tissue, integrate and function – the hope is that this eventual tissue replacement will have a long-lasting and regenerative effect in the retina.
Research has progressed to such an extent that a number of early phase clinical trials are underway, and some have already reported results on their use for retinal diseases such as advanced AMD or Stargardt’s disease. It’s worth noting that these studies have involved the surgical subretinal transplantation of retinal pigment epithelial cells derived from embryonic pluripotent stem cells (3),(4),(5). Initial results have been encouraging: the procedure appeared to be tolerated in most eyes and some patients experienced improved visual function afterwards. But subretinal cell delivery is not without danger. Every manipulation of the retina risks damage, and the intervention needs to be more curative than the manipulation is damaging. There is also a big safety issue; because these cells are allogeneic, prolonged systemic immunosuppression is required to avoid rejection of the transplanted cells. The problem is that systemic immunosuppression was not tolerated in all subjects in these studies. Having said all of this, there may be an alternative source of allogeneic stem cells for treatment that can sidestep this issue.
Bone marrow as a stem cell source
Adult bone marrow is a source of therapeutic stem cells, and it’s one that’s actively being explored for the treatment of a number of diseases, including those affecting the retina. Figure 1 shows the two principal bone marrow stem cell types that are currently under investigation: mesenchymal stem cells (MSCs) and hematopoietic stem cells (HSCs). HSCs in humans express the CD34+ cell surface protein, making them easy to identify by immunohistochemistry (6), but MSCs are more easily harvested and expanded by bone marrow cell culture – and have already been evaluated in animal models as stem cell therapy for retinal diseases. There is debate over whether MSCs differentiate into cells beyond mesodermal origin – but what they can definitely do is produce factors that induce a paracrine protective effect on surrounding tissues (6),(7),(8),(9),(10),(11),(12),(13),(14). Another appealing advantage of MSCs is that they can be autologous or allogeneic without immunosuppression’ without immunosuppression. By contrast, HSCs do not readily expand in culture and must be harvested from the bone marrow mononuclear cell fractions by positive selection based on cell surface markers (which, in humans, is principally CD34) (14).

Figure 1. Types of stem cells isolated from bone marrow. Mesenchymal stem cells are easily cultured and expanded from bone marrow aspirate. Human hematopoietic stem cells can isolated by the cell surface marker, CD34. Human hematopoietic stem cells can isolated by the cell surface marker, CD34.
Both the intravitreal and subretinal MSC administration routes have been explored in animal models of retinal degeneration, with both displaying a neuroprotective effect on the degenerating retina with minimal engraftment. Studies also show subretinal MSC administration may be the more effective route of administration for the treatment of retinal degeneration (13),(14). However, intravitreal MSC injection would be the simpler and easier way of administering cell therapy; unfortunately, this approach can result in the cells clumping in the vitreous cavity. In vivo retinal imaging has shown fibrovascular proliferation that results in significant complications like tractional retinal detachment (14), raising safety concerns about this route of administration. To date, there are no published clinical data using MSCs for the treatment of retinal disease, although multiple Phase 1 and 2 clinical trials have been conducted for non-ocular conditions without safety concerns (14).
Human bone marrow CD34+ cells contain mostly HSCs. These cells can differentiate into various cells of various blood cell lineages, and may also have paracrine regenerative effects (6),(14). The CD34+ cells include endothelial progenitor cells (EPCs) that are mobilized into the peripheral circulation in response to tissue ischemia and are thought to play an important role in tissue revascularization (6). In mouse models of ischemic retinal vasculopathy, CD34+ cells have been shown to home in on the damaged retina and retinal vessels and secrete factors that promote tissue repair and regeneration (15),(16). The CD34+ HSCs may play an important role in patients with retinal disease. For example, CD34+ HSC levels are elevated in the systemic circulation of patients with exudative AMD, and it is possible these cells play a role in the physiologic repair response to the disease state (17). By contrast, defects in the homing capability of CD34+ cells in peripheral blood of diabetic subjects have been observed, and it’s thought that this plays a role in the pathogenesis of a number of diabetic complications, including retinopathy (18)(19)(20).
Our work
We have shown that intravitreal administration of human CD34+ cells from bone marrow into the eyes of NOD-SCID mice with acute retinal ischemia-reperfusion retinal injury results in not only long-term incorporation of the human cells in the retinal vasculature but also the apparent normalization of the retinal vasculature (21). Why NOD-SCID mice? We chose them as their innate immunodeficiency makes them incapable of rejecting the human CD34+ cells. The safety profile observed in the NOD-SCID mice was excellent, with no ocular or systemic adverse effects being associated with the administered CD34+ cells injected into the vitreous; the CD34+ cells themselves remained viable and detectable within the retinal vasculature for over six months. The fact that this study demonstrated a favorable long-term safety profile with this route of cell therapy lead to the FDA issuing Investigational New Drug Application (IND) clearance to explore this cell therapy in a clinical trial.
We’ve also used a systemically immunocompromised mouse model of retinal degeneration to investigate the effect of intravitreally-administered human bone marrow-derived CD34+ stem cells on inherited retinal degeneration (22). In this study, Pde6brd1/rd1 mice were used, as they display a rapidly progressive severe retinal degeneration with loss of electroretinographic (ERG) signals by four weeks of age. The mice were immunosuppressed pharmacologically with tacrolimus and rapamycin, which were delivered continuously using an implanted ALZET pump. Following immune suppression, we administered either GFP (green fluorescent protein)-labeled CD34+ cells harvested from human bone marrow, or saline by intravitreal injection. The mice underwent in vivo retinal imaging to visualize the cells in the eye. Simultaneous scanning laser ophthalmoscopy and optical coherence tomography were used. Then, the mice were euthanized at either one or four weeks after the injection of stem cells for histological and microarray analysis of the retina.
As with the previous study, the safety profile was excellent and no ocular or systemic adverse effects were observed. The GFP-labeled CD34+ cells appeared to home in rapidly into the retinal surface and seemed viable over the entire study duration of the four-week period after injection. Microarray analysis of the gene expression changes in the retinae of these mice after CD34+ cell injection demonstrated altered expression of more than 300 genes – predominantly those regulating photoreceptor function and maintenance as well as apoptosis. These findings support the concept that the CD34+ cell therapy can affect the degenerating retina at multiple levels via multiple pathways, similar to the effects of MSCs described above (22),(23),(24). We proposed that the observations were best explained by a paracrine effect of the CD34+ cells as we observed no direct incorporation of human cells into the degenerating photoreceptor layer in these mice.
Clinical promise
Our group also initiated a Phase I clinical trial investigating intravitreal autologous CD34+ cell therapy for retinal disease under an IND cleared by the FDA. The CD34+ cells were isolated from bone marrow of patients with ischemic or degenerative retinal disorders and administered autologously (NCT01736059). The bone marrow aspiration and intravitreal cell injection were performed in-office under local anesthesia on the same day, no systemic immunosuppression was used, and the CD34+ cells were isolated from the mononuclear cell fraction of the bone marrow aspirate under Good Manufacturing Practice conditions. The first six patients included two patients with Stargardt’s disease, two patients with AMD, one patient with retinitis pigmentosa and one patient with a combined central retinal artery and vein occlusion (CRAO/CRVO). As this is a Phase 1 clinical trial, all subjects had advanced permanent vision loss in the study eye at enrollment. After six months, four of the six eyes showed visual acuity improvements of two or more lines during the study follow-up period (25). The most dramatic improvement in vision was achieved in the patient with CRAO/CRVO, where that pathogenesis of vision loss is more acute and ischemic rather than progressive and degenerative. No ocular or systemic complications were recorded in any of the study subjects.
The results of the Phase I clinical trial showed the promise that CD34+ cells have for retinal regeneration and further investigation is planned. The advantages of this approach to cell therapy are obvious: CD34+ cells are relatively simple to obtain from bone marrow and can be used autologously without the need for systemic immunosuppression. Intravitreal cell delivery is technically simple and may be an effective route of cell delivery for the treatment of retinal disorders based on preclinical studies (13). The paracrine effects of these cells on damaged retina may allow this cell therapy to have a broad clinical application that may be therapeutic for both degenerative and ischemic retinal diseases – think of the potential it might have to treat the baby-boomer generation with age-related retinal disease. The safety profile of this cell therapy has been excellent thus far but, clearly, larger clinical trials are needed to further characterize the safety and efficacy of this cell therapy. Given that some serious ocular adverse effects have been reported in individuals receiving unregulated cell therapies for vision loss, it’s critical for patient safety that the proper characterization and isolation of cells in bone marrow is performed before intraocular administration (14).
The ultimate goal of this area of research is to develop a therapeutic treatment for patients with vision loss from retinal disorders that are currently untreatable without compromising patient safety. If this can be achieved, the consequences for patients, medicine, and society could be profound.
Elad Moisseiev is a vitreoretinal surgeon in the Department of Ophthalmology, Tel Aviv Medical Center, in Tel Aviv, Israel. He is a faculty member of the Sackler School of Medicine at the Tel Aviv University, and completed his ophthalmology residency training at the Tel Aviv Medical Center, and vitreoretinal surgery fellowship at the University of California Davis, Sacramento, CA, USA.
Susanna Park is a Professor of Ophthalmology at the University of California Davis, Sacramento, CA, USA. She is a vitreoretinal surgeon and clinician scientist who has conducted research on bone marrow stem cell therapy for retinal disease for over ten years.
The authors thank the following collaborators at the University of California Davis: Jan Nolta and Gerhard Bauer of the Institute for Regenerative Cures for the stem cell isolation, Robert Zawadzki for retinal imaging and Zeljka Smit-McBride for microarray analysis. The authors report no conflicts of interest.
- RR Bourne et al., “Causes of vision loss worldwide, 1990-2010: a systematic analysis”, Lancet Glob Health, 1: e339–349 (2013). PMID: 25104599.
- JB Jonas et al., “Visual impairment and blindness due to macular diseases globally: a systematic review and meta-analysis”, Am J Ophthalmol, 158, 808–815 (2014). PMID: 24973605.
- SD Schwartz et al., “Embryonic stem cell trials for macular degeneration: a preliminary report”, Lancet, 379, 713–720 (2012). PMID: 22281388.
- SD Schwartz et al., “Human embryonic stem cell-derived retinal pigment epithelium in patients with age-related macular degeneration and Stargardt’s macular dystrophy: follow-up of two open-label phase 1/2 studies”, Lancet, 385, 509–516 (2015). PMID: 25458728.
- WK Song et al., “Treatment of macular degeneration using embryonic stem cell-derived retinal pigment epithelium: preliminary results in Asian patients”, Stem Cell Reports, 4, 860–872 (2015). PMID: 25937371.
- SS Park, “Cell therapy applications for retinal vascular diseases: diabetic retinopathy and retinal vein occlusion”, Invest Ophthalmol Vis Sci, 57, ORSFj1–ORSFj10 (2016). PMID: 27116667.
- J Xia et al., “Bone marrow mesenchymal stem cells stimulate proliferation and neuronal differentiation of retinal progenitor cells”, PLoS One, 8, e76157 (2013). PMID: 24098776.
- P Duan et al., “Human bone marrow stromal cells can differentiate to a retinal pigment epithelial phenotype when co-cultured with pig retinal pigment epithelium using a transwell system”, Cell Physiol Biochem, 31, 601–613 (2013). PMID: 23635912.
- C Huang et al., “Combination of retinal pigment epithelium cell-conditioned medium and photoreceptor outer segments stimulate mesenchymal stem cell differentiation toward a functional retinal pigment epithelium cell phenotype”, J Cell Biochem, 113, 590–598 (2012). PMID: 21948619.
- X Sun et al., “E13.5 retinal progenitors induce mouse bone marrow mesenchymal stromal cells to differentiate into retinal progenitor-like cells“, Cytotherapy, 13, 294–303 (2011). PMID: 20979443.
- LL Yang et al., “Differentiation of human bone marrow-derived mesenchymal stem cells into neural-like cells by co-culture with retinal pigmented epithelial cells”, Int J Ophthalmol, 3, 23–27 (2010). PMID: 22553511.
- TV Johnson et al. “Identification of retinal ganglion cell neuroprotection conferred by platelet-derived growth factor through analysis of the mesenchymal stem cell secretome”, Brain, 137, 503–519 (2014). PMID: 24176979.
- A Tzameret et al., “Transplantation of human bone marrow mesenchymal stem cells as a thin subretinal layer ameliorates retinal degeneration in a rat model of retinal dystrophy”, Exp. Eye Res, 118, 135–144 (2013). PMID: 24239509.
- SS Park et al., “Bone marrow stem cell therapy for retinal dysfunction”, Prog Eye Ret Res [Epub ahead of print]. (2016). PMID: 27784628.
- RJ Medina et al., “Molecular analysis of endothelial progenitor cell (EPC) subtypes reveals two distinct cell populations with different identities”, BMC Med Genomics, 3, 18 (2010). PMID: 20465783.
- P Madeddu, “Therapeutic angiogenesis and vasculogenesis for tissue regeneration”, Exp Physiol, 90, 315–326 (2005). PMID: 15778410.
- Y Yodoi et al., “Circulating hematopoietic stem cells in patients with neovascular age-related macular degeneration”, Invest Ophthalmol Vis Sci, 48, 5464–5472 (2007). PMID: 18055794.
- CJ Loomans et al., “Endothelial progenitor cell dysfunction: a novel concept in the pathogenesis of vascular complications of type 1 diabetes”, Diabetes, 53, 195–199 (2004). PMID: 14693715.
- S Brunner et al., “Correlation of different circulating endothelial progenitor cells to stages of diabetic retinopathy: first in vivo data”, Invest Ophthalmol Vis Sci, 50, 392–398 (2009). PMID: 18719083.
- X Liu et al., “Endothelial progenitor cells (EPCs) mobilized and activated by neurotrophic factors may contribute to pathologic neovascularization in diabetic retinopathy”, Am J Pathol, 176, 504–515 (2010). PMID: 19948824.
- SS Park et al., “Long-term effects of intravitreal injection of GMP-grade bone-marrow-derived CD34+ cells in NOD-SCID mice with acute ischemia-reperfusion injury”, Invest Ophthalmol Vis Sci, 53, 986–994 (2012). PMID: 22247454.
- E Moisseiev et al., Intravitreal administration of human bone marrow CD34+ stem cells in a murine model of retinal degeneration”, Invest Ophthalmol Vis Sci, 57, 4125–4135 (2016). PMID: 27537262.
- T Meyerrose et al., “Mesenchymal stem cells for the sustained in vivo delivery of bioactive factors”, Adv Drug Deliv Rev, 62, 1167–1174 (2010). PMID: 20920540.
- M Gnecchi et al., “Paracrine action accounts for marked protection of ischemic heart by Akt-modified mesenchymal stem cells”, Nat Med, 11, 367–368 (2005). PMID: 15812508.
- SS Park et al., “Intravitreal autologous bone marrow CD34+ cell therapy for ischemic and degenerative retinal disorders: preliminary phase 1 clinical trial findings”, Invest Ophthalmol Vis Sci, 56, 81–89 (2014). PMID: 25491299.
Elad Moisseiev is a vitreoretinal surgeon in the Department of Ophthalmology, Tel Aviv Medical Center, in Tel Aviv, Israel. He is a faculty member of the Sackler School of Medicine at the Tel Aviv University, and completed his ophthalmology residency training at the Tel Aviv Medical Center, and vitreoretinal surgery fellowship at the University of California Davis, Sacramento, CA, USA.
Susanna Park is a Professor of Ophthalmology at the University of California Davis, Sacramento, CA, USA. She is a vitreoretinal surgeon and clinician scientist who has conducted research on bone marrow stem cell therapy for retinal disease for over ten years.
The authors thank the following collaborators at the University of California Davis: Jan Nolta and Gerhard Bauer of the Institute for Regenerative Cures for the stem cell isolation, Robert Zawadzki for retinal imaging and Zeljka Smit-McBride for microarray analysis.