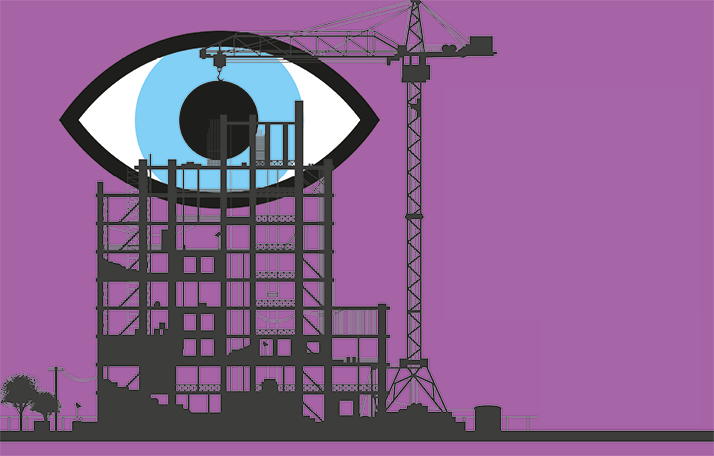
Retinal degeneration is a lifelong process. Today’s imaging technology can detect the earliest signs of diseases like AMD in people in their mid-forties, and advances like adaptive optics-based imaging systems might push that point back by a decade or more. The problem lies at the other end of the spectrum: people in their senior years with degenerating and degenerated retinae. Improved nutrition might slow progression, and there are a number of drugs in development that might retard degeneration, but ultimately, if people are to regain some of their visual function, you’re looking at having to rebuild the retinal structures they’ve lost – or implanting artificial retinal prostheses.
Rebuilding an entire retina is a tall order, but perhaps it’s not altogether necessary. The retinal pigment epithelium (RPE), situated between the choroid and the neural retina, is central to retinal function. The RPE acts as a “metabolic gatekeeper” between photoreceptors and the choriocapillaris, supporting the function of the highly metabolically-active photoreceptors and dealing with their waste. Both the RPE and Bruch’s membrane suffer cumulative damage over a lifetime – which is thought to be one cause of AMD in susceptible individuals (1). So can the RPE be replaced? Possibly. Autologous transplantations of the RPE and choroid have been successfully performed, but with a high surgical complication rate (2), they’re not deemed feasible, rather they help prove the concept. Stem cells might be the source of that replacement, both human-derived embryonic and induced pluripotent stem cells (hESC and iPSC, respectively). It’s possible to transplant individual RPE cells in a suspension into the subretinal space by forming a bleb retinal detachment with these cells, but there are two principal concerns with this method: limited cell survival and the possibility of abnormal cell architectures being formed in vivo (3). But there could be a way of overcoming these limitations: growing these cells on a scaffold and delivering that to the retina has been shown in animal models to enhance cell survival and direct differentiation to appropriate retinal cell types. Boris Stanzel, a consultant ophthalmologist at Bonn University Eye Hospital and lecturer at the University of Bonn, is one of the pioneers of using stem cells to grow RPE on a scaffold followed by transplantation into the retina. The Ophthalmologist spoke with Boris about his groundbreaking research.
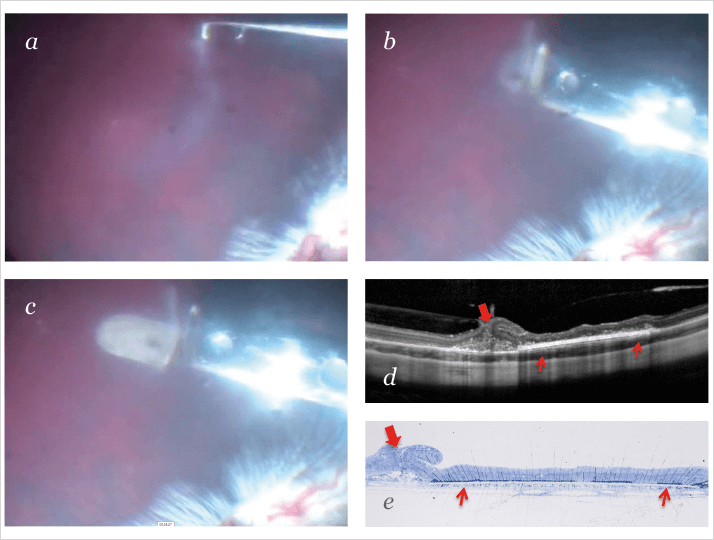
How do you prepare RPE cells for transplantation?
The implants I use combine RPE cells and a carrier that the cells attach to. The rationale for doing this is that it can satisfy many needs at once. For instance, if you use the right substrate as a carrier, you can grow and control the cells on that substrate and achieve the behavior that you want. Once you’ve accomplished that, you need to make sure that your material is easily handled during surgery, meaning that the surgeon has an implant that can be safely and precisely placed into the subretinal space. Thinking beyond implantation, you have to utilize the material properties of the carrier to optimize integration of the RPE implant into the subretinal space, which is crucial for the implant to work. In other words, you can create a functionalized carrier that is not only a substrate – not only a platform for bringing the cells into the subretinal space – but also intelligent, so that the wound healing essentially provides a seamless, scar-free integration into the subretinal space.What substrates are you using to make the carriers?
I’ve been working with several materials. These substrates must be porous, but that isn’t the only consideration; the surface features – particularly nanotopography – also seem to control cell behavior. A lot of RPE culture substrates are composed of materials that are completely smooth, but it turns out that this is far from ideal for RPE cells. An ideal substrate would be slightly rough, sort of “nano-roughish” material. The easiest one I’ve found to work with so far has been polyethylene terephthalate, which is a porous ultrathin smooth membrane and actually the same material that is being used in London by Pete Coffey’s group for their embryonic stem cell-derived RPE project (bit.ly/petecoffey). I remember talking about it with them back in 2006: “Oh, we both have the same material, that’s fun!” We’ve also been working with biodegradable materials like gelatin (4) and nanofiber-based materials (5,6), both of which are attractive, but we’re not sure yet if we’ll be using a biostable cell carrier going forward or if we’re going to use a biodegradable carrier.What are patients most likely to favor?
I think from an emotional perspective, patients would prefer the biodegradable approach. You don’t want to have something permanent in your body if you can avoid it. Think of, say, a stent in cardiac surgery, or an artificial knee; patients anticipate it wearing out. If you have a biodegradable material, you have to ensure that, over the long-term, the cells will produce enough of their enabling matrix to reconstitute, at least to some degree, clinically functional subretinal architecture.How do you fabricate the substrates you use?
The nanofiber materials that we use are created with a process called electrospinning. Surprisingly enough, this is a technology that has been around for 30 or 40 years. It’s been used in filter systems like the ones you find in air conditioners. Then, some 10 years ago or so, people found out that cells love to grow on this stuff. We were the first ones to try to put RPE cells onto that substrate. We were amazed at the way we were able to revive and rejuvenate elderly human RPE cells that nobody thought could be grown properly in culture. And of course, if you place a cell carrier into the subretinal space, you expect that, to some extent, the surrounding cells will integrate with those on the carrier. So having a proper material that allows the cells to grow and behave the way they’re supposed to is a great advantage.But there’s more to regenerating the retina than just implanting the RPE on the substrate.
If you’re talking about regenerating the retina, then you need to consider the photoreceptor cells as well. I don’t know whether you could do something like combined RPE-photoreceptor transplants; I think that’s quite a complicated approach. The process of transplanting photoreceptors is a very different one. They really rely on the cells migrating into the retina, which is fundamentally different to RPE replacement. People are also considering cell carrier materials for photoreceptor transplantation, but those materials differ vastly from the ones used with RPE; at the moment, people are mostly talking about hydrogel-type materials. We have also worked with hydrogels for RPE transplantation in the past, but not directly with the materials that are being considered for photoreceptor transplants. For photoreceptor transplants, you want to have single cells at a certain immature stage, and this is different from RPE cells, where the retinal pigment material needs to be fairly mature in order to be clinically functional. So that’s somewhat of a difference in approach – which is why I think it might be very challenging to do a combined RPE and photoreceptor transplant.How do you determine when to do the transplant?
If you’re aiming at RPE transplantation or replacement, you need to define your therapeutic window. Transplantation will not work in patients who have gone beyond a certain level of neural retinal regeneration, and I think we’re now in the lucky position that we can very precisely tease apart who will and will not be a candidate for transplant. In other words, RPE transplantation is not the magic bullet that will cure everything. This is very key for people to understand, because nowadays there’s talk about RPE transplants being used in geographic atrophy (GA). The essential question is: where and when? It makes no sense to put an RPE transplant into an area of significant GA – in other words, where the RPE cells have been gone for years. It makes sense to put the RPE transplant on the edge of this atrophy, because that is where you can otherwise expect the atrophy to spread, and so that’s where you can make a difference and prevent atrophy progression. I had the chance to speak with an eminent retinal surgeon, Bernd Kirchhof, who is director of the Department of Vitreoretinal Surgery at the Uniklinik Köln and who has performed over 300 such transplants. I have great respect for his work and one of the things he told me has stuck with me; he said, “If you want to put in an RPE transplant, you avoid patients who have a large geographic scar.” The problem is that you just want to get the retina off in order to bring your transplant in. But if you want to put your transplant into the area that has been lost to RPE cell death, if you try to lift the retina off, you tear it apart – and that’s a problem.Are there other interventions in the pipeline for patients with GA?
For GA, there are already several very intriguing technologies. Perhaps the biggest breakthrough is the complement factor D blocker, lampalizumab, which is the first drug to significantly retard GA progression. Sure, the disease still progresses, but if a patient with macula-sparing GA can get a shot that slows its progression from 3 mm² a year to 1 mm², then that patient will love you. I see potential in this sort of complement-inhibiting technology, but patients hate the monthly shots. The real attractiveness will come from a one-time procedure, perhaps using something like a viral vector. The key is to find a delivery material that works in the eye. One company uses polyethylene glycol (PEG) nanoparticles, but PEG isn’t biodegradable, so it might accumulate and eventually cause side effects. Gelatin is a very attractive biomaterial, too, but in the retina it’s pro-inflammatory and pro-angiogenic, so despite the fact that it’s widely used in tissue engineering, I’m not yet sure it would work well in the eye. I feel that there are much more elegant integration methods than we’ve been able to use so far, and those might allow us to administer therapy in a single shot, which would make the treatments much better – at least from the patients’ perspective. One, as yet not openly discussed future possibility, would be to bridge patients with disease-retarding pharmacologic therapies and then, along the course, offer cell replacement to them, if deemed suitable.Do you ever see this becoming a routine surgery for good candidates?
I think the advantage of having a tissue-engineered RPE transplant is that it will make the procedure much simpler, faster and safer than it currently is. Nowadays if you’re trying to put in an RPE transplant, you first need to isolate it in the periphery and then “swirl it” across the vitreous cavity, which can release enough RPE cells to cause proliferative vitreoretinopathy. Finally, you shove the transplant underneath the macula and squash it, so there’s a likelihood of inducing fibrotic reactions around the transplant. None of that is likely to happen with the more modern technology, largely because you’re not going to swirl the transplant around in the vitreous because it’s protected in your instrument. The transition period where it’s exposed to the vitreous briefly – or not at all – may not really matter. It could be that, once the implantation procedure and wound healing strategies are well sorted out and refined – say, 10 or 20 years down the line – this might be an implantation technique that could be done in roughly 30 minutes. I could even imagine that one day RPE transplantation could be done by robotic surgery, which would greatly enhance consistency and reproducibility, much like cataract and refractive surgery nowadays is moving toward robotic surgery with a femtosecond laser. The first attempts at doing retinal surgery in a virtual, three-dimensional environment has shown that, basically, the surgeon doesn’t even have to be in the operating room any more – they can control everything out of their office. So 20 years down the line, RPE transplantation may well be a routine procedure as well.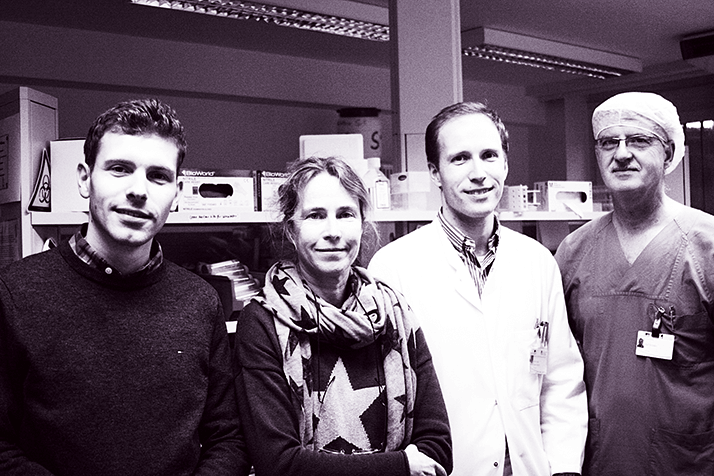
Could transplantation potentially help patients with inherited genetic defects of the RPE, perhaps with gene therapy?
I think the beauty of the RPE cell nowadays is that we have learned that we can generate it from many different cell sources. The new cells would lack the genetic defect because if you use iPS cell technology, you can isolate a skin or blood biopsy from the patient, create RPE cells in a dish and correct the gene defect (if it can be corrected). People are very excited about iPS technology in RPE transplantation; the first clinical trial is now launching in Japan, and the next one – which I’m actually involved in – will (hopefully) be launching at the National Institutes of Health in the US in 2018. Another patient population that I find very interesting is patients who have suffered toxic RPE damage, for instance, through chloroquine maculopathy. Patients who have received a certain cumulative dose of hydroxychloroquine – which is now more common than chloroquine – start to experience symptoms and visual field defects, and you can see subtle changes in the RPE. That may be the time to transplant, because they still have photoreceptor function. You get in, remove the area of degenerating RPE, and replace them with healthy cells.How much of the RPE can you restore?
There are several technologies out there that address that issue, but I think at the moment the most elegant solution to the size – and to the eventual visual field – is what’s now loosely termed the “taco approach.” from the London and California Project for Blindness. The implant has a lip; a specialized instrument grabs that lip, pulls the implant into a hollow tube and rolls it like a taco – just like a corneal graft surgery. That gives you more surface area and it touches fewer cells. The downside of that approach is that, in order for it work, you have to enter the subretinal space. The other way of approaching the problem is to actually make a larger scleral incision, so you can then use a wider instrument that allows you to have a longer, wider implant. You shoot that into the subretinal space from outside the retina. This allows you to largely avoid entering the subretinal space and potentially either damaging the retina or the choroid and causing a massive subretinal hemorrhage. Once you run into a subretinal hemorrhage, you’re probably “done”, and the patient will be blind. A third option to approach this, would be to make a peripheral 180º temporal retinotomy, gently detach and fold over the neural retina, place an implant of desired size in the macular area and reattach the retina. Taken together, both instrument design and surgical factors are the current challenges that limit the area of the RPE we will be able to replace. I think it’s hard to say which approach will win out in the future. I think at the moment, the field is still in its infancy, and all those brave enough to go into clinical trials will be learning along the way. I don’t think anyone can say right now, “This is the technology that’s going to win the race.” I think we are all in the same boat, so we should all pull together.So your interest in this lies in scientific and medical advancement?
Yes primarily, and if the business part of it comes along the way, then that’s just a nice bonus. I think that’s a nicer way of going about it, because then you’re pursuing what you like, rather than money. If you’re doing it to pay the bills, you aren’t really going to be a paradigm changer. Take cataract surgery as an example. Cataract surgery 60 years ago was very crude. When Harold Ridley realized that PMMA splinters embedded in pilots’ eyes were inert in the eye, and did not induce an immune response, that knowledge enabled us to make intraocular lenses (IOLs) out of it. Where are we today? We can perform clear lens extraction, we have multifocal IOLs, and refractive surgery is a huge field – so we have come very far since then. We’re just starting with stem cell clinical trials now, so imagine where we will be in 20 years! That’s the fascination; that’s what drives me to do the research I do.References
- S Binder et al., “Transplantation of the RPE in AMD”, Prog Retin Eye Res. 26, 516–54 (2007). PMID: 17532250. RE MacLaren et al., “Autologous transplantation of the retinal pigment epithelium and choroid in the treatment of neovascular age-related macular degeneration”, Ophthalmology, 114, 561–70 (2007). PMID: 17324698. J Kundu et al., “Approaches to cell delivery: substrates and scaffolds for cell therapy”, Dev Ophthalmol. 53, 143–54 (2014). PMID: 24732768. BV Stanzel et al., “Subretinal delivery of ultrathin rigid-elastic cell carriers using a metallic shooter instrument and biodegradable hydrogel encapsulation”, Invest Ophthalmol Vis Sci. 53, 490–500 (2012). PMID: 22167099.