Translational Research: Innovate Horizontally
Bridging the “Valley of Death” of expertise for medical device development
At a Glance
- Disruptive innovation is almost never a small evolutionary change to the status quo
- There are two approaches – vertical translation and horizontal translation: neither is easy, but…
- Horizontal translation provides a better template for medical devices to follow than the traditional, big pharma vertical approach
- IOLs, corneal laser surgery and OCT imaging are all examples of what can be achieved when horizontal translational approaches are adopted.
When it comes to technological innovation, the medical device industry is a peculiar place. In most other fields of development, the critical factor for successful development and commercialization of a technology is the technology itself: it will succeed on its own merits. But if the technology has a medical application, a number of additional factors come into play: regulatory requirements, reimbursement policies, and ultimately user acceptance can all have a decisive impact on an innovation’s destiny and dictate its financial success.

Figure 1. Vertical technology path: Innovator is pushing the technology upwards through multiple levels of increasing complexity to the user. At the end of the journey he might face the fact that there is no application for the technology or invention.
Disruptive medical device innovation
Harvard Business School’s Professor of Business Administration, Clayton Christensen, and his co-authors Jerome Grossman and Jason Hwang, proposed the disruptive innovation model (1) – a particularly helpful method of understanding technology adoption across many domains. It states that one can expect an underperforming – but promising – technology to be adopted first in the least demanding market niche. Multiple development iterations over time improve the product, which can result in an expanded market presence – and the product’s market success is determined by its fitness for purpose. In the most extreme case, such a product can eventually completely seize the market.
The difficulty we have with products with medical applications is getting it to the market in the first place (let alone finding the least-demanding niche or undergoing the iterative improvements that will hopefully lead to a market-conquering device). In addition to the regulatory and reimbursement scheme hurdles that need to be cleared, if a product deviates from an established medical procedure, it will meet additional resistance that needs to be overcome. But disruptive innovation is almost never a small evolutionary change to the status quo.
There are two distinct pathways to innovation: the first is the classical bottom-up, or vertical translation model (Figure 1), where the developed technology is actively “pushed” to the potential users; the second is the horizontal translation model (Figure 2), where a technology is “pulled” from other domains to provide a potential solution. Though each pathway type is a crucial step towards efficient translational research, it’s important to understand the specific challenges of each process.
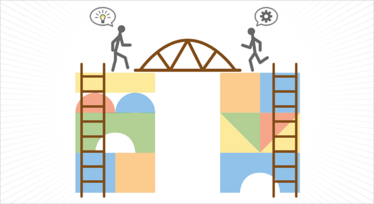
Figure 2. Horizontal technology path: innovation is pulled between domains at comparable levels of complexity, which helps generate better mutual understanding between innovator and user.
The vertical approach
When it comes to medical devices, you can’t rely on the classical bottom-up innovation model, which is comprised of a basic research phase (usually performed in academia) where the fundamental physical or biological mechanisms are investigated for a better understanding of the underlying theory. This phase is typically funded by public research programs such as the US National Institutes of Health (NIH) or the European research councils. Funds are awarded for research that satisfies the conditions of originality and the robustness of the proposed methodology. But after that, it’s assumed that commercial, privately-funded institutions will step in at the applied research stage. As the developed technology is actively pushed to potential users, this strategy is known as a “push” type of technology adoption. It’s evident that this product development method is increasingly failing to generate useful health innovations. The pharmaceutical industry is a great example. Research councils have supported countless discoveries in genomics and biology, and have funded initial breakthroughs in such potentially game-changing fields as nanotechnology and stem cell therapies… but the translation of these basic findings to the development of new drugs with clinical applications has been, as one Nature Editorial put it, “disturbingly limited” (2). The same piece stated, “The uncomfortable truth is that scientists and clinicians have been unable to convert advances in biological basic pure research into therapies or resolve why these conversion attempts so often don’t succeed.”
In the 1990s, even the US Congress recognized that there was lack of substantial industry involvement in medical innovation, and supported a doubling of the NIH’s health research budget – as long as that research had the principal objective of improving the health of the nation (3). This was the foundation for a translational research strategy intended to promote the links between basic laboratory investigations, clinical investigations, applications of clinical trial outcomes in community settings, and decisions about public health policy. Practically, this meant funding those prioritized multi- and trans-disciplinary approaches for translating research discoveries into clinical practice in order to improve health outcomes (3) – and it ended up creating a new discipline: translational science.
The success of the translational science approach depends on the ability to establish appropriate (and cooperative) relationships between those with bench-science expertise in academia and those who understand unmet clinical needs and how to apply the product “at the bedside” – the healthcare provider. This creates a “continuum of innovation” (3) from basic to applied science and back again. In some respects, though, the paradigm applied (traditional or translational) doesn’t matter in terms of innovation: advances are driven from the bottom up, towards the user – it’s known as the “vertical translation pathway” (Figure 1).
It’s no surprise that the average time it takes for the pharmaceutical industry to develop a drug is 10–15 years. “Bench to bedside” is a slow process, and there are a number of reasons inherent to the industry for this.
The first: every step along the path from the lab bench towards a marketable drug is associated with an increase in complexity as it progresses from molecular understanding through to clinical application in humans (4,5) – so lengthy innovation cycles are understandable. It’s costly too; in the early 2000s, the average cost of drug development – including the cost of failures – was estimated at $1.2 billion (6), and today’s costs are even greater.
Complexity is only part of the story. Tech transfer from academia to industry for product development and manufacture has historically been fraught with complications. Differences in aims, working cultures, timetables, expectations and outcomes between both communities have often led to considerable difficulties in communication and collaboration – and serve to create gaps along the knowledge transfer path (7).
To understand why this is the case, you need to understand that the factors motivating academia and industry are disparate. Both act to improve the lives of patients. Universities reward their researchers for basic discoveries and the publication of results. Applied, commercial research needs to financially benefit the organization funding the work – and they need patents to commercialize their intellectual property, meaning there’s a considerable need for secrecy within the organization before they reveal their discoveries to the world and their competitors in a patent.
Innovations then have to pass through the “Valley of Death” to make it to commercial reality; this is where a project has progressed past the point where public funding is available… but private funding is still out of reach. Why? Risk-averse investors typically prefer to invest at a later stage of development, when most of the technical risks are minimized and returns on investment can be better predicted (6). Many technically and scientifically brilliant concepts have failed at this stage.
When scientists drive innovation, they may start from a point that makes clinical application difficult. They often follow a “backwards” process where the innovation itself justifies the research, but this method of technology adoption – and the resulting need to push the technology to its potential users – can lead to a product whose clinical outcomes are unable to meet regulatory requirements.
Lab-derived innovations need to prove their functionality and usability before they can enter the clinic – but without the expertise of people actively involved in clinical work, this is a difficult task. If new technologies aren’t up to the challenge, regulatory and reimbursement procedures can also suffer. As Thomas Fogarty once said, “what a doctor wants, what they say they want, what they need, and what they will pay for, are all different things” (7).
These challenges, taken together, make the innovator-driven approach to drug development comparable to a climb up a very steep mountain through multiple levels of technology sophistication on the pathway from bench to bedside. However, this is not the only technology translation pathway. There are several examples of successful innovations in ophthalmology that moves technology between domains of comparable level of sophistication: “horizontal translation” (Figure 2), which is usually driven by healthcare practitioners.
The horizontal approach
In the realm of medical device technology, though, there’s a better way to accomplish technology transfer. Whereas pharmaceuticals are usually designed for a specific disease, medical devices typically apply technology developed in other areas (chip manufacturing, photochemistry, optics, electronics, and so on) that might not be regulated to the same degree (8). In this case, innovators involved in clinical application can initiate a “horizontal translation” of the technology. For instance, a clinician who spots a problem in his practice may search for solutions in other domains; when he identifies a potential solution, he pulls the technology to the medical domain. In this example, the innovator is responding to the pull of technology, as opposed to the push of strategy characteristic of vertical translation. Because the clinician is both the initiator and the end-user in this context of innovation, he is more likely to take into account scientific and economic considerations that make commercial success more feasible (6,9,10).
Many new technologies have found their way into ophthalmology by horizontal translation. Much of this success was achieved through multiple iterations or adaptations of existing technologies that have resulted in products with clear ophthalmic health benefits.
The First IOL
The inventor of intraocular lenses (IOLs), Harold Ridley, was a British ophthalmic surgeon in the Second World War. He was dissatisfied with the cataract surgery of the era because it left patients aphakic and dependent on poorly performing spectacles. While treating pilots in World War II, he noticed that splinters of acrylic from aircraft cockpit canopies did not trigger rejection alike glass splinters did when lodged in patients’ eyes – which led him to propose the use of artificial lenses to correct cataracts. Lacking appropriate chemical knowledge, Ridley joined forces with scientists John Pike of Rayner and John Holt of ICI to develop a suitable form of acrylic (11). Five years later, Ridley implanted the first IOL into an aphakic woman with 20 D of hyperopia. Following surgery, the patient was 14 D nearsighted. Though the power of the IOL was clearly wrong – and power calculation was corrected and refined for subsequent procedures – Ridley proved that an artificial lens could be successfully implanted into the eye. From concept to product, the innovation of the first IOL took only 5 years to establish the beginnings of what turned out to be a new norm for cataract surgery.
Corneal laser surgery
In 1981, Rangaswamy Srinivasan discovered that an ultraviolet excimer laser could precisely etch living tissue without damaging the surrounding area. He called this phenomenon ablative photodecomposition (APD). In 1983, Srinivasan collaborated with Steve Trokel to develop APD to etch the cornea, which resulted in the refractive procedure we now know as LASIK. Since its introduction, millions of people have taken advantage of LASIK surgery to reduce their dependency on corrective lenses.
Optical Coherence Tomography
In 1988, Fercher adopted low-coherence interferometry, used to characterize optical fibers, for in vivo eye length measurements (12). The adoption of this and other interferometric techniques for ophthalmology eventually led to the development of a tomographic technique, known as optical coherence tomography (OCT), for in vitro ocular tissue imaging. The availability of low-cost components designed for the telecommunications industry contributed to the simplicity of adapting the technology for clinical experiments, and groups involved in the development of OCT were linked with clinical research institutions from the beginning.
Implementation of the horizontal translation pathway
Insight is only the beginning of innovation – but the hard work required to turn an idea into a finished product is often left out of success stories. The innovation pathway involves several development stages with continual validation (“Are we building the right thing?”) and verification (“Are we building it right?”). Those stages are:
- Exploratory investigations,
- Technical and clinical proof-of-concept,
- Product development,
- Product launch and life cycle management,
- Post-market surveillance and clinical investigations,
- Product improvements.
Academia usually focuses on basic science and exploratory investigations; it’s industry’s job to create commercial success by establishing products with long-term uses and beneficial outcomes. The technical and clinical proof-of-concept stage (B), which involves a combination of skills to demonstrate the feasibility of an idea, links the two. The advantage of horizontal innovation is that feasibility can be evaluated relatively inexpensively during the early exploratory stage (A).
A crucial part of the validation process is asking: Does the device fulfill its clinical purpose according to the intended use with beneficial outcomes and acceptable risks? In the past this might include either a literature review of equivalent devices at the end of the development process, but in the future will increasingly require a clinical demonstration of efficacy and safety. Working with a clinical practitioner passionate about the technology, who can find an appropriate application in ophthalmology, allows the demand for successful validation and verification to be user-driven; otherwise, it will be technology-driven and its wide range of potential uses may result in poor clinical utility. The most successful medical device products focus compelling technology on a very specific clinical need, considering the intended use of the device, its expected clinical success, its potential risks and patient safety, and its usability from a clinical perspective.
Once user needs are fully understood, the next step is to design the device and specify its system requirements. During the engineering and testing phases, interim reviews of the design verify that it meets those needs, while a final validation test at the end of the development phase ensures that the device has been built correctly and that it fulfills the user requirements. Then it can progress to legal review (for instance, in Europe, under the medical device directives), a step that shouldn’t be underestimated, as it can substantially influence development costs and timelines. Finally, the new device can enter clinical use – at which point the main concerns become manufacturing, marketing, regulatory activities related to post-market surveillance and ongoing product improvements.
Even in a horizontal translation pathway, companies only embrace technology that has already successfully passed technical and clinical proof-of-concept, as it maximizes the revenue-to-risk metric. Innovators may be able to perform, at most, some of the stage A requirements, but then find themselves in the “Valley of Death” between stages A and B. For a confident innovator, this is an excellent opportunity to develop a technology as a private startup company until it can be taken over by a large industrial player. Financially, this “Valley of Death” can be bridged with the help of private investment or venture capital – but it’s difficult to acquire the expertise and finances needed to develop and execute clinical trials and validate a proof-of-concept within the lifespan of a startup. Support from a partner with required expertise can play a decisive role in translating the technology from innovator to industry.
It’s not easy, but it’s viable
Horizontal translational research is no easy task, but despite this, it’s a more viable strategy than the questionable eventual success of vertical translation. In recent decades, great health improvements suggest that the benefits of medical technology far outweigh its costs. Yet healthcare expenditure on medical technology remains relatively small and more or less constant over time. Bearing in mind increasing budget constraints, patient and clinical expectations, and the shift from private to public funding, there’s a rising need for high-quality products with observable “bedside” outcomes. The promotion and support of horizontal translational research is the key to creating reliable products with clear benefits for patients, clinicians and society.
The classical duo of academia and industry can benefit from an experienced third party to bridge the gap between their two worlds.
Michael Mrochen is the founder of IROC Science AG, Zurich, Switzerland, a company specializing in translational research projects for medical devices in the field of ophthalmology and vision care. Pavel Zakharov, Mark S. Talary and Daniel Boss are consultants at IROC Science AG.
- C. M. Christensen, J. H. Grossman, and J. Hwang, The Innovator’s Prescription. McGraw Hill (2009).
- Editorial. “Hope in translation,” Nature, 467, 499 (2010). doi: 10.1038/467499a.
- UHealth, “What Is Translational Research?” Accessed September 30, 2014.
- J. Cunha-Vaz and E. Zrenner, “Special Report Translational Research in Ophthalmology - A European Perspective,” Eur. Ophthalmic Rev., 5, 13–15, (2011).
- EurovisionNet, “White Paper on Translational Research in Ophthalmology and Vision Sciences in the European Union” (2012). accessed November 26, 2014.
- Pharmaceutical Research and Manufacturers of America, “Biopharmaceutical Research Industry Profile” (2013). Accessed November 26, 2014.
- T. R. Kucklick, “The Medical Device R&D Handbook”, CRC Press (2006).
- WHO, “Introduction & Medical Devices,” in Medical devices: managing the mismatch (2010). http://apps.who.int/medicinedocs/documents/s17704en/s17704en.pdf, accessed November 26, 2014.
- J. Wilkinson, “The medical technology industry in Europe,” (2011), http://bit.ly/EUCOMED, accessed November 26, 2014.
- WHO, “Public health needs,” in Medical devices: managing the mismatch, (2010). http://apps.who.int/medicinedocs/en/d/Js17704en/, accessed November 26, 2014.
- H. Ridley, “Intra-ocular acrylic lenses; a recent development in the surgery of cataract”, Br. J. Ophthalmol., 36, 113–22, (1952).
- A. F. Fercher, K. Mengedoht, and W. Werner, “Eye-length measurement by interferometry with partially coherent light”, Opt. Lett., 13, 186–8, (1988).
Michael Mrochen is most recently known for his pioneering work on corneal collagen crosslinking, but this is not his first innovation. Mrochen’s research with Theo Seiler (qv) led to the development of both wavefront-guided and wavefront-optimized LASIK, which has transformed outcomes, minimized errors and made LASIK a safer and more predictable procedure.