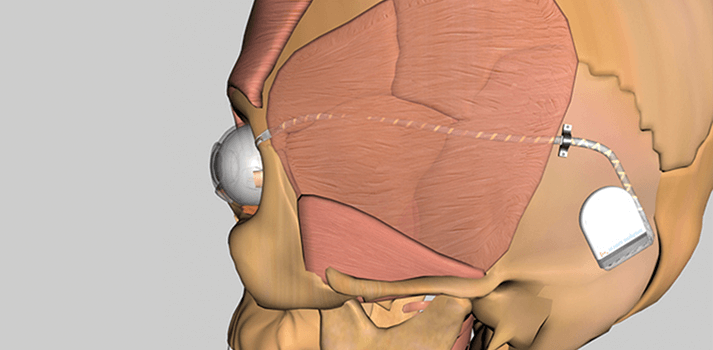
- Biomedical implants have the potential to restore vision to patients with retinitis pigmentosa
- Clinical trials with epiretinal and subretinal implants have generated positive results
- Two devices has received regulatory certification in Europe
- The most advanced biotechnological approach uses subretinal microchips
Retinitis pigmentosa (RP) describes a heterogeneous group of genetic disorders that cause progressive peripheral vision loss and night vision difficulties, eventually leading to central vision loss. It affects one in every 3–4,000 people in the Western world and is among the leading causes of progressive sight loss. Symptoms usually appear in childhood and increase in severity over time.
The pharmaceutical industry is currently investigating various approaches to slow the progression of the disease, but all still require many years of clinical study, and these potential therapies are not expected to restore vision to patients with late-stage RP. The final frontier is using artificial vision to treat completely blind patients. Retinal implants represent the most promising advance in vision restoration via two main approaches – subretinal and epiretinal microchips.
The main difference between these two approaches is the location of the chip. Epiretinal implants sit on the retina addressing the neuronal output. Subretinal implants (see Figure 1a) are placed beneath the retina replacing photoreceptor function at the input side – specifically in the macular region – and work along with the natural processing of images in the human brain (1). Suprachoroidal implants are available, which are also implanted underneath the retina, but from the scleral side, meaning that the choroid and pigment epithelium are located between the chip electrodes and retinal neurons. The risks associated with this surgical technique are lower, but only a few suprachoroidal implantations have been made, since spatial resolution is inferior with these devices.
In recent years, many researchers have come to agree that positioning implants subretinally, where the light-sensitive photoreceptor cells were located before they degenerated, may be the most favorable approach (2). With the image receiver positioned within the eye, vision is restored as the eye scans from left to right and up and down, allowing for the immediate focus and recognition of items in the patient’s field of vision. The location in between retinal layers also provides excellent mechanical stability, meaning that the chip’s connection with neurons is unlikely to loosen over time. At present, subretinal microchips (3) can contain considerably more pixels than are available in epiretinal (4) devices (1,500 versus 60), thereby providing higher spatial resolution and greater contrast.
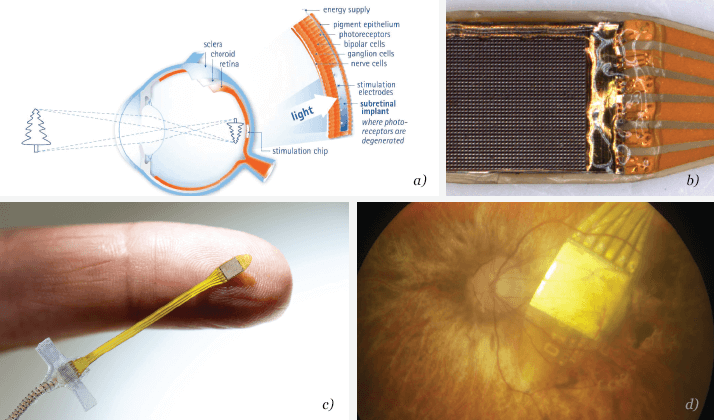
Development and testing
The consortium that developed the subretinal implant (see Figure 1b) started in 1995 at the Institute of Ophthalmic Research, part of the Centre of Ophthalmology at the University of Tübingen in Germany, in cooperation with the Institute for Microelectronics Stuttgart (IMS) and the Natural and Medical Sciences Institute (NMI) in Reutlingen. After eight years of preclinical work a company, Retina Implant AG, was founded in 2003 in nearby Reutlingen to produce the retinal implant chips and to sponsor clinical trials.The Alpha IMS subretinal implant was designed to imitate the eye’s natural processing of light. The team designed a 3 x 3 mm2 array containing 1,500 tiny light-sensitive photodiodes, each with an amplifier that augments the light-evoked current and forwards it to an adjacent electrode (see Figure 1c). This chip is placed on the tip of a very thin foil that carries gold wires to supply power and provide signal control of the amplifiers. At the other end of the 25 mm-long foil, there is a connection pad with a thin cable that leads to a receiver coil that is around the size of a large coin. Apart from a thin cable that leads from the battery pack kept in the patient’s pocket (that leads to a transmitter coil behind the ear) nothing is visible externally.
During implantation, the surgeon makes a tiny window into the globe of the eye within the orbit and gently advances the light-sensitive array under the retina towards the fovea, the area of optimal vision (see Figure 1d). The microchip’s thin power connection foil (also under the retina) leaves the globe through the window, which is closed after this. It is then linked to the cable that is routed (under the skin) to a place behind the ear where the coil is placed subcutaneously (see diagram at start of article). The chip is activated when the patient puts another coil externally above the subdermal coil. The external coil remains in place via a small magnet, and the pairing of both coils powers the device and enables the transmission of signals through the skin. The implant is powered by a mobile phone-sized battery pack that can be easily carried around by the patient. The pack features two control buttons – for contrast and for brightness – enabling the sensitivity of the subretinal micro-photodiode array to be adjusted depending on the available light.
A key advantage of this approach is that there is no camera outside the body. The light-sensitive chip sits in the ideal position – on the retina – where photoreceptors had previously been before they deteriorated. The chip and its array of 1,500 electrodes move with the gaze of the eye, meaning that the patient can view objects in the whole volume in front of them, at a ±50° angle both horizontally and vertically. Furthermore, natural microsaccades help to constantly refresh the image and to avoid image fading.
To date, 36 patients have received this subretinal microchip in clinical trials across Europe and Asia. The company’s first clinical trial began in 2005 at the University Eye Clinic of Tübingen, Germany, where eleven patients received the implant – which at that time, was still cable-bound – for three months. The results were encouraging (3); the most successful recipient went from being able to identify vertical and horizontal lines on a computer screen to form letters into words; in one extraordinary moment, he corrected the spelling of his name! He recognized a banana and could distinguish between a fork, knife and spoon. Placement of the implant beneath the fovea clearly provided optimum visual results, allowing this and other patients to recognize objects and facial expressions as well as read words and see dots on a pair of dice.
A second clinical trial began in 2010 in Germany. For the first time, patients in this trial were allowed to keep the chip implanted permanently, enabling researchers to assess the benefits in everyday life outside of the laboratory. Initial results indicated that over the three-to-nine month observation period, functional vision was restored in the majority of the patients implanted (5). Additionally, the visual acuity of two patients surpassed the highest visual resolution of any patient in the first trial, and three patients were able to read letters spontaneously. Patients also reported the ability to distinguish objects such as telephones and read signs on doors.
With the momentum of positive results from the clinical trials to date, and the generation of $18 million in venture capital funding, trial sites outside of Germany were added, including sites in Oxford, London and Hong Kong. In June 2013, the Alpha IMS device received a CE mark, enabling it to be marketed across the EU. This represented the first European regulatory certification for the company, and the decision marks an important milestone in the journey to make subretinal microchip technology accessible to the wider patient community. It provides clinicians across Europe with a chance to offer their patients a new pathway to regain vision. This epiretinal approach now has both CE mark and FDA approval and is therefore available for use in the European Union and the United States.
Looking ahead
Research to advance the subretinal microchip technology continues, in order to build on the success of the two clinical trials. Plans are underway to commence a clinical trial in the United States, the results of which will form the basis for a regulatory application to the FDA.These results of epiretinal (4) and subretinal (3, 5) trials prove that the technology can offer patients who are blinded by photoreceptor degeneration the freedom of having visual perception outside of the laboratory setting. New comprehensive clinical trial programs will allow us to learn more, and by listening to feedback from patients participating in the trial, we can customize the device to suit their needs.
Eberhart Zrenner is the Distinguished Professor of Ophthalmology at the Institute of Ophthalmic Research at the Centre of Ophthalmology, University of Tübingen, Germany. He is also an initiator of the SUBRET consortium and one of the founders of Retina Implant AG.
References
- E. Zrenner, “Artificial vision: solar cells for the blind,” Nat Photonics, 6, 344-345 (2012). K. Mathieson et al., “Photovoltaic retinal prosthesis with high pixel density,” Nat. Photonics, 6, 391–397 (2012). E. Zrenner et al., “Subretinal electronic chips allow blind patients to read letters and combine them to words,” Proc. Biol. Sci., 278, 1489–97 (2011). M. S. Humayun et al., “Interim Results from the International Trial of Second Sight’s Visual Prosthesis,” Ophthalmology, 119, 779-88 (2012). K. Stingl et al., “Artificial vision with wirelessly powered subretinal electronic implant alpha-IMS,” Proc. Biol. Sci., 280 (1757):20130077 (2013).