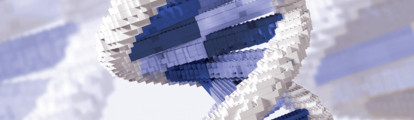
Gene Editing: The Next Frontier in Ophthalmology
Imagine being able to edit, delete or replace defective genes. It’s happening in research labs, and will soon head to the clinic
At a Glance
- Gene editing is one of the hottest topics in biomedical research today, as it holds the potential to ‘correct’ genetic mutations and restore a normal phenotype
- There are three main gene editing methods currently in use: CRISPR/Cas9, ZFNs and TALENs, with CRISPR/Cas9 being the most commonly used gene editing tool today
- Of particular interest in ophthalmology is the treatment of CEP290-associated Leber congenital amaurosis using CRISPR – with clinical trials planned to commence next year
- If clinical trials show that gene editing procedures are safe and effective, then this opens up the potential to treat a great number of ocular genetic disorders
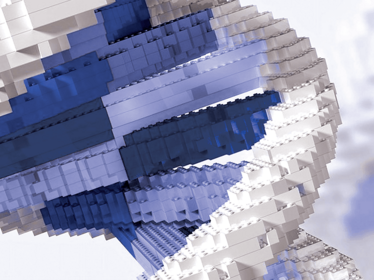
There has been considerable lay media coverage of gene editing techniques, with one in particular, CRISPR/Cas9, receiving most of the attention. ARVO designated gene editing as one of the “hot topics” of their 2015 annual meeting in Denver, and already one company is developing a CRISPR/Cas9-based treatment of the inherited retinal disease Leber congenital amaurosis (LCA), with plans to commence clinical trials of the therapy next year. But what is CRISPR/Cas9 anyway – and are there alternatives?
A gene editing overview
Put simply, gene editing is the insertion, replacement or removal of DNA from the genome using artificially engineered nucleases, often termed “molecular scissors.” The principal therapeutic application of this would be the modification of a mutant gene’s DNA sequence to the normal “wild-type” form.
In contrast to gene therapy, which introduces a copy of a gene without removing or replacing the existing DNA, gene editing is able to replace or eliminate existing genetic material. By creating specific double-strand breaks in the DNA using restriction enzymes, gene editing technologies are able to “cut out” sections of DNA, and exploit the mechanisms of double-strand break repair to introduce template DNA and insert new genetic material (1). Currently, there are three popular technologies used to achieve this: CRISPR/Cas9, ZFNs, and TALENs (see Sidebar “Gene Editing Technologies: The Three Main Players”).
The currently available approaches to gene editing deploy molecular guides that bind specific DNA sequences and then use nucleases to generate double-strand breaks, which trigger DNA repair. ZFNs and TALENs have been used for several years, but now, the (relative) newcomer CRISPR/Cas9 has displaced them as the research tool of choice because of its speed, ease of use, and lower cost. According to the market research firm MarketsandMarkets, the global market for gene editing will top US$3.5 billion by 2019 (3), with CRISPR accounting for the largest and fastest-growing segment.
Why CRISPR/Cas9?
As reported by Stephen Rose (4), chief research officer of The Foundation Fighting Blindness, “CRISPR/Cas9 is different from the gene therapies currently in human studies, which involve gene replacement – i.e., delivering copies of a whole new normal gene to replace the defective copies causing vision loss. In contrast, CRISPR/Cas9 is a gene ‘cut-and-paste’ technology. It works like a molecular scissors to cut out the mutated portion of the gene and inserts a healthy piece of DNA.”
“Think of it this way: Let’s say your car won’t start. You have two options: Buy a new car or fix the part that’s causing the problem. Getting a new car is like gene replacement. Getting a new part to fix the problem – for example, a battery, starter or fuel pump – is like CRISPR/Cas9,” he adds.
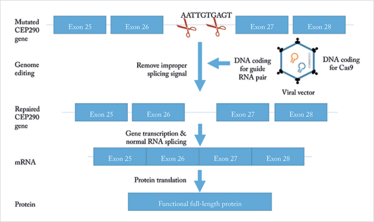
Figure 1. Editas hopes to treat mutated CEP290 by introducing a viral vector containing CRISPR into the eye, which will delete an improper splicing signal, allowing for translation of a functional protein.
Rose also raises some important points on the pros and cons of gene editing. One big advantage of CRISPR/Cas9 is getting around the problem of delivering large genes. One example is USH2A – a gene that encodes for a 1,551 amino-acid protein called usherin, which is an important component of basement membranes in the retina and plays a central role in the development of the eye. It’s a large gene and several mutations in the gene have been identified that can lead to the development of retinitis pigmentosa (RP) 39 and Usher Syndrome 2A. The problem with a traditional gene therapy approach is that the gene is too large to fit into any currently available viral vector – but with CRISPR/Cas9, that’s now achievable, with the technique being able to delete and insert DNA sequences of at least 65 kilobases. The technique may also represent a simpler approach to treating diseases, in which delivering a whole new gene is not necessary. Simply the act of shutting down or repairing the bad gene may be enough to save vision, as is the case with autosomal dominant RP – and CRISPR/Cas9 can perform this.
The downside to CRISPR/Cas9, at least at the moment, is that it often doesn’t work efficiently enough to restore vision, and it can have unwanted, off-target effects on healthy genes. But progress is being made, and researchers are continuing to improve the effectiveness and specificity of the gene editing technique.
Getting CRISPR into the clinic
An example of a company working to bring CRISPR/Cas9 to human trials is Editas Medicine, which was founded about three years ago by Jenifer Doudna (no longer associated with the company) of UC Berkeley, California, and Feng Zhang of MIT/Broad Institute, Massachusetts, along with other collaborators, to investigate the treatment of a broad range of genetically driven diseases.
Of the various diseases Editas is working on, the one of interest to ophthalmologists is LCA. In particular, a mutation of the CEP290 gene leads to LCA10 – a rare retinal disease that, according to Jean Bennett, Director of Advanced Retinal and Ocular Therapeutics at the University of Pennsylvania’s Medical School, affects only about 600 people in the US. Bennett, who is not involved in the Editas study, says that “the target that they have selected is fantastic; it has all of the right characteristics in terms of making a correction easily.”
CEP290-associated LCA is challenging to treat, because like USH2A, it’s a gene that is too large for the adeno-associated virus vectors currently being used in clinical trials of gene-replacement therapies for retinal disease. It’s for this reason that Editas’ approach uses CRISPR/Cas9 to correct defective copies of CEP290.
Speaking in early November at the EmTech Conference at MIT in Cambridge, Massachusetts (5), Editas Medicine’s CEO Katrine Bosley said the company hopes to start a clinical trial in 2017 to treat LCA using CRISPR/Cas9. If Editas’ plans move forward, the study would likely be the first to use CRISPR/Cas9 to edit the DNA of a person in a clinical trial.
Editas chose the disease in part because it is relatively easy to address with CRISPR/Cas9, Bosley says. The exact mutation in the gene is known, and the eye is easy to reach with genetic interventions. However, there are still questions about how well gene editing will work in the retina, and what side effects could potentially be caused by unintentional changes to DNA.
The company plans to deliver the CRISPR/Cas9 technology using gene therapy – this will involve injecting a “soup” of viruses loaded with the DNA that codes for all of the proteins required to produce CRISPR/Cas9 in the retina. Bosley says that in order to treat LCA, the company intends to delete about 1,000 DNA bases from the CEP290 gene in patients’ photoreceptor cells. In vitro experiments suggest that this intervention enables the gene to function correctly again. Bosley admits that Editas still needs to test the approach further in the lab, and then in animals, before a human study can be undertaken.
By targeting an exceptionally rare illness, Editas may have an easier time getting a treatment tested and approved by regulatory bodies. However, the eventual cost of such a treatment could be extraordinarily high, given the small number of people who would need it. Bennett says only around 3,000 Americans have LCA, and about 20 percent of those have the form being targeted by Editas.
Future directions
Gene editing, and CRISPR/Cas9 in particular, has only been used for a relatively short time – a few years – to change or remove defective genes, so there are relatively few research programs underway at the moment that address its use in ophthalmology. A literature search returns only a few references to the current use of CRISPR/Cas9 techniques in treating ophthalmic diseases:
- Donald Zack, Johns Hopkins University, is developing CRISPR/ Cas9 for treating autosomal dominant RP.
- Edwin Stone, University of Iowa, is developing a different CRISPR/ Cas9 approach for the correction of defective retinal genes, including USH2A (which codes for a form of Usher Syndrome), MAK (male germ-cell associated kinase), and RHO (Rho kinase inhibitors, for treating glaucoma).
- Benjamin Bakondi et al., Regenerative Medicine Institute, Cedars-Sinai Medical Center, Los Angeles, are using CRISPR/Cas9 to demonstrate the reversal of retinal defects in an animal model of RP.
- David Courtney et al., School of Biomedical Science, University of Ulster, Coleraine, Northern Ireland, are using CRISPR/Cas9 to treat ocular corneal dystrophies in animals.
- Most recently, Bayer and CRISPR Therapeutics have entered into an agreement to work together to discover, develop and commercialize new breakthrough therapies based on CRISPR/Cas9 technology to cure blood disorders, blindness, and congenital heart disease. According to the Wall Street Journal, the eye disease they aim to treat will be Stargardt disease (6).
The next frontier in ophthalmology?
The ability to edit and correct – or even remove and replace – defective genes in the eye appears likely to become reality over the next few years. The pace of progress with this technology is rapid; the first clinical trial of gene editing for the treatment of inherited retinal disease could be underway as soon as next year. If successful, this could lead the way for gene editing for the treatment of ophthalmic genetic diseases, providing ophthalmology with a new way to restore vision to those who have lost it – and historically had little hope of ever recovering it.
Irv Arons reports on new drugs and devices for the treatment of retinal diseases. A former consultant to the ophthalmic and medical laser industries with management consultants Arthur D. Little for 25 years, Irv ran his own company, Spectrum Consulting, for 11 years before his retirement.
Gene Editing Technologies: The Three Main Players (2)
CRISPR/Cas9 – clustered regularly interspaced short palindromic repeats; CRISPR associated protein 9
This technique integrates infecting viral DNA among the palindromic CRISPR sequence repeats. Bacterial enzymes then transcribe the CRISPR sequence and its interspersed viral sequences into a “guide” RNA. The guide RNA associates with the Cas protein to bind and induce a double-strand break at the integration site of the viral DNA in the chromosome, snipping it out.
ZFNs – Zinc finger nucleases
ZFNs were the first of the systems to be identified and described in the scientific literature. A zinc finger is a small protein motif, discovered in the African clawed frog (Xenopus) in 1987, that folds into an oblong shape when it binds zinc atoms. The zinc finger domain binds to a specific DNA sequence that, when joined to a DNA-cleaving domain, forms a scissor-like molecular tool.
TALENs – transcription activator-like effector nucleases
The third system, TALENs, resembles ZFNs in that the cleavage domains of both ZFNs and TALENs include the endonuclease domain of the restriction enzyme Fok1. The DNA binding domain of TALENs is 33 to 34 amino acids taken from the plant pathogen Xanthomonas, differing only at residues 12 and 13, which can be tweaked to add binding specificity. Researchers began developing TALENs as gene-editing tools in 2009; they are considered more efficient than ZFNs.
- JS LaFountaine et al., “Delivery and therapeutic applications of gene editing technologies ZFNs, TALENS, and CRISPR/ Cas9”, Int J Pharm, 494, 180–194 (2015). PMID: 26278489.
- R Lewis, “Gene editing trials poised to expand, but hurdles remain”, Medscape Medical News, November 30, 2015. Available at: bit.ly/riklewis. Accessed January 11, 2016.
- Markets and Markets, “Genome editing market worth $3,514.08 million by 2019”, (2015). Available at: bit.ly/1R8Y9VX. Accessed January 5, 2016
- Foundation Fighting Blindness, “ARVO 2015 highlights: a cut-and-paste approach to fixing retinal-disease gene”, (2015). Available at: bit.ly/1H0V5kF. Accessed January 5, 2016.
- MIT Technology Review, “CRISPR gene editing to be tested on people by 2017, says Editas”, (2015). Available at: bit.ly/1kvhLWm. Accessed January 5, 2016.
- The Wall Street Journal, “Bayer in venture with gene-editing startup”, (2015). Available at: on.wsj.com/1Pflnq0. Accessed January 5, 2016.
Irv Arons’ Journal is a well-read blog that reports on new drugs and devices for the treatment of retinal diseases, including age-related macular degeneration (AMD). Until his retirement, Irv was a consultant to the ophthalmic and medical laser industries, working for management consultants Arthur D. Little for 25 years and running his own company, Spectrum Consulting, for 11 years.