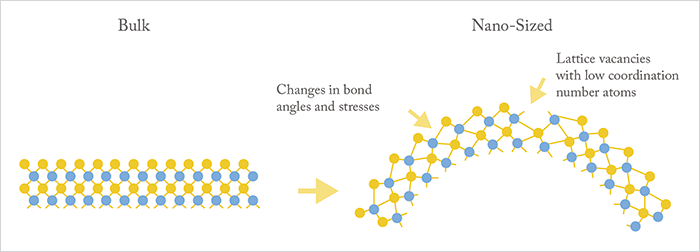
- Current treatment options for ocular cancer lead to substantial, or even complete vision loss – and aren’t always successful
- It’s time to explore new approaches to improve both visual and survival outcomes for patients
- Nano-sized semiconductors can be used to synthesize toxins inside tumor cells, while potentially sparing vision
- Initial in vivo and in vitro studies show promise, but further studies of efficacy and toxicity are needed
Semiconductors are everywhere. The semiconductor-based microchip became a big thing in the late 1970s, and they’re in everything now, from the watch on your wrist, to the TV on your wall, and even in the security tag on a packet of razor blades. Now there’s a new application with huge potential – cancer therapy. We can produce nano-sized semiconductors that, under illumination by visible light, synthesize toxins inside tumor cells. Because tissue in the eye is easily illuminated, semiconducting nanoparticles look to be an extremely promising therapeutic intervention in ocular cancer, and may spare patients from the vision loss that normally occurs with traditional anticancer treatments.
Preserving vision with nanometer precision
Let’s establish why the unmet need exists first. Ocular cancer is usually managed by enucleation and radiation, unarguably a substantially invasive method, which inevitably leads to substantial or complete vision loss – and even then it’s not always successful in managing the disease. There’s a clear need for new tools that not only enable oncologists to better manage ocular cancer, but also to improve patients’ vision and survival. One of the current treatment options for certain ocular cancers is photodynamic therapy (PDT). It works – suboptimally. Today’s photosensitizers are destroyed by the reactive oxygen species (ROS) produced during illumination. What my colleagues and I propose is a new generation of photosensitizers that are based on semiconducting nanoparticles: WO3/Pt nanoparticles (1),(2),(3). The crucial difference is that these nanoparticles do not photobleach after hours of illumination, and this puts the treatment period in the hands of the physician, not the chemistry of the photosensitizer. I like to think of WO3/Pt nanoparticles as the world’s sharpest scalpels, because of their small size – and the even smaller distances that their product, hydroxyl radical, can diffuse after synthesis. In other words, this approach produces the same toxins as radiation treatment, but within a nanometer-sized region. Clearly, it could hold the promise of precise treatment, which spares healthy tissue and improves patient outcomes.Curiouser and curiouser
As Alice discovered in Wonderland, things are not what you might expect at very small sizes – the bulk properties of a crystal do not necessarily apply to nanocrystals. This change in scale has a number of implications. Nanoparticles have a very high radius of curvature (Figure 1), and this is important as it alters bond angles and lengths between the atoms. This can cause crystal faults or vacancies on the nanoparticle surface; these tiny defects in the crystal can act as catalytic sites – and that’s something we can exploit. To give you an example, let’s take the surface of a metal oxide nanoparticle. It has a vacancy on its surface thanks to a missing oxygen atom, and the pocket this makes (with its altered chemistry) could then act like the active site of a conventional enzyme and perform chemical catalysis – such as the production of hydroxyl radicals. Further, each nanoparticle has many of these catalytic sites, multiplying the overall rate of hydroxyl radical production, so even a tiny particle can produce a big amount of product.A radical approach
WO3/Pt nanoparticles contain two components: the tungsten trioxide crystal acts as a semiconducting photoanode, and the platinum acts as a nano-electrode photocathode (see Figure 2). What this means is that when the nanoparticles are exposed to light, current flows from the semiconductor photocathode to the platinum crystals. In doing so, the nanoparticles grab electrons from nearby organic molecules (especially electron donors such as NADPH), which then accumulate at the Pt photocathodes, and go on to reduce oxygen to hydroxyl radicals – a very highly ROS. By using NADPH to produce cytotoxic ROS, the particles mimic the NADPH oxidase of phagocytic cells. In other words, we use inorganic crystals exhibiting biological catalytic abilities to enhance or replace a normal anti-tumor response.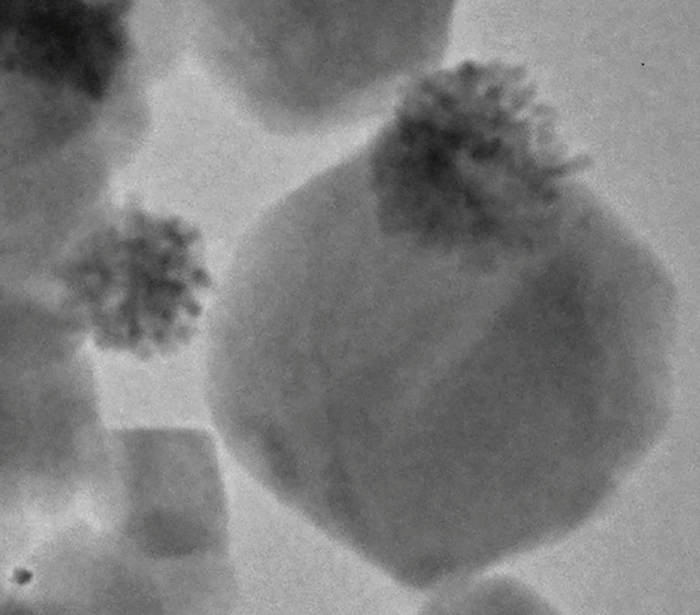
Figure 2. Appearance of a WO3/Pt nanoparticle. Tufts of platinum crystals (the co-catalyst) are easily seen at one side of the nanoparticle.
Creating ROS to kill cancer cells isn’t a new idea – it’s an approach that’s common to many other anti-cancer strategies like radiotherapy and many chemotherapies. But we all know the drawbacks of these approaches: collateral damage; ROS kills normal cells too. Retinal cells are especially sensitive to ROS, and it’s thought that ROS may also contribute to retinal diseases such as glaucoma, macular degeneration, and diabetic retinopathy. But the beauty of the nanoparticle approach is that it can be targeted to tumor cells with covalently-attached folate molecules, where they manufacture ROS solely within those cells, minimizing damage to neighboring cells. Let’s compare and contrast this approach with PDT. PDT dyes do bind tumor cells, but with less specificity, and you’re already aware that they rapidly photobleach, limiting the period of effective treatment. With inorganic nanostructures, there’s no concern over photobleaching, the substrates (oxygen and organic compounds) are readily available within cells, and they’re effective over a broad range of pH values. What this all means is that these nanoparticles can generate high levels of hydroxyl radicals (roughly 10 million per nanoparticle each hour) to kill the tumor cells they’re bound to.
Short-circuiting normal metabolic pathways
The process of hydroxyl radical production involves the removal of electrons from biomolecules, which has the side-effect of destroying the constituents of the tumor cell. In addition to this lysosome-like activity, nanoparticles also utilize the cell’s metabolic pathways to drive tumor cell death. Remember, an important substrate for the nanoparticle is the cellular electron carrier NADPH. When a tumor cell is exposed to ROS, it increases its hexose monophosphatase activity in order to synthesize more NADPH. But this enzyme is also required to make reduced glutathione – which is used to manage toxins. Further, NADPH supplies electrons to aldehyde reductase, which also helps to deflect an oxidative attack by destroying aldehydes formed downstream from hydroxyl radicals. Finally, NADPH is used by quinone reductase in an attempt to minimize damage caused by hydroxyl radicals and lipid radicals buried within the membrane. Just one hydroxyl radical produced by the WO3/Pt nanoparticle can lead to the formation of many lipid hydroperoxides, which are disruptive to lipid bilayers, and ultimately toxic aldehyde compounds like 4-hydroxy-nonenal, which has been shown to induce apoptosis in tumor cells. Ultimately, the beauty of this approach is that, by short-circuiting normal metabolic pathways, the nanoparticles not only produce hydroxyl radicals, they weaken the tumor cell’s ability to respond to the attack.Efficacy and toxicity
There’s a large gap between what works in theory and what works in practice. We’ve shown, using multiple in vitro assays, that these WO3/Pt nanoparticles induce apoptosis in tumor cells in the presence of light (Figure 3). But what about in vivo? Animal experiments have shown that WO3/Pt nanoparticles significantly (P<0.00001) extend the lifetimes of tumor-bearing mice (2) – and that this effect required the combination of all three components: light, WO3 and Pt to induce tumor killing, as suggested by the mechanism outlined in Figure 4. When we tested folate-modified, tumor-directed WO3/Pt nanoparticles in a model of breast cancer metastasis to the anterior chamber, we found that not only did the nanoparticles selectively accumulate at the tumors, but that (once again) illumination resulted in ocular tumor cell apoptosis.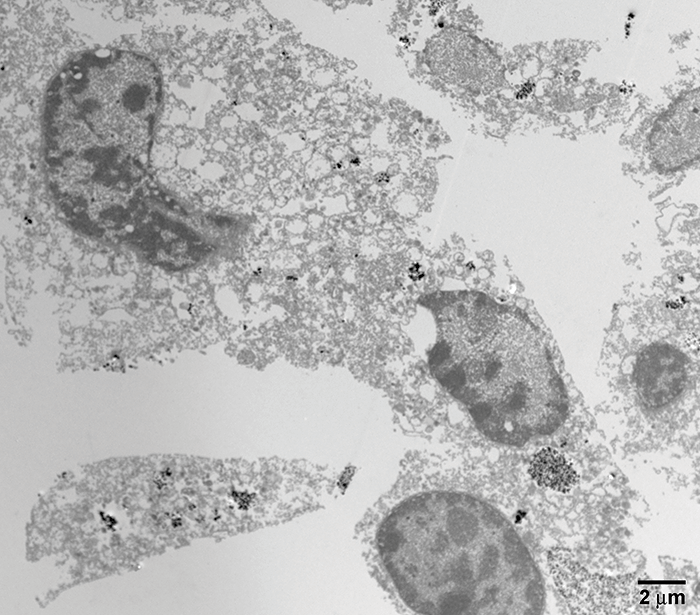
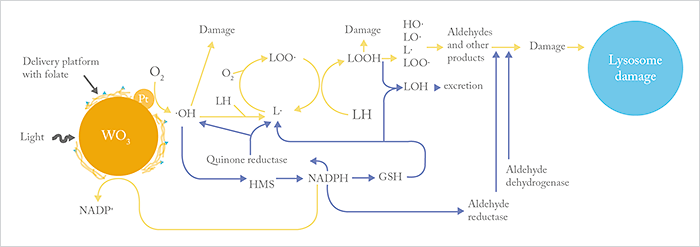
Knowing that the nanoparticles show efficacy in vivo is one thing, but we also need to know their potential for harm – and we do realize that further toxicology studies in animals are necessary to address this. Although monomeric WO3 and WO3 nanoparticles have little or no toxicity (4),(5), the toxicity of WO3/Pt nanoparticles has not been established. Our early studies at clinically relevant WO3/Pt nanoparticle doses suggest that they have no effect on ocular structures. Moreover, the safety of these nanoparticles is also supported by the fact that the LD50 of monomeric WO3 (1059 mg/kg), which can be cleared by the kidney, is higher than those of aspirin (~200 mg/kg) and caffeine (between 150–200 mg/kg).
Helpfully, some of the properties of these nano-sized semiconductor particles can be exploited in future toxicology studies: the X-ray scattering properties of metal/metal oxide nanoparticles could be used in conjunction with micro-CT scans to map the presence and movement of nanoparticles over time within animals, and it should be possible to use WO3/Pt nanoparticles tagged with infrared probes to monitor their location within animals. These approaches would clearly complement the ongoing conventional toxicology studies of WO3/Pt nanoparticles.
Big changes with small particles
There’s a great deal of interest in nanotechnology across all of medicine for both diagnostic imaging and therapeutic applications – their use, conjugated with a cell-targeting ligand, as a drug or gene delivery vehicle is under intense investigation. Our approach of replacing ineffective biological immune responses with robust inorganic crystals, I believe, holds a lot of potential. But we need to tread carefully. These are very new compounds, and we need to understand the toxicology of the nanoparticles in greater detail before they might see clinical use.There may also be room for improvement. Yes, the fact that these nanoparticles scavenge NADPH reduces the tumor’s ability to deflect an oxidant attack via reduced glutathione production and quinone and aldehyde reductase activity. But aldehyde dehydrogenase, which is greatly overexpressed in cancer stem cells, is unaffected by the nanoparticles, and this may account for the ability of tumor cells to regrow in our model of breast cancer metastasis. The logical next step to try to improve anti-tumor responses would be combining this nanotherapy with conventional aldehyde dehydrogenase inhibitors. This might not be a “magic bullet,” but it should hopefully make for a powerful weapon in the ocular oncologist’s arsenal. Semiconductors aren’t just used to deal with bits and bytes; in my opinion, they’re also one of the most promising approaches on the horizon for the treatment of ocular cancer. But in many respects, both are acting in a very binary manner: microchips deal in on/off scenarios; ones and zeros. When it comes to our nanoscale semiconductors, what’s at stake is tumor cell status: dead or alive? Howard Petty is a Professor at the Kellogg Eye Center, University of Michigan School of Medicine in Ann Arbor, Michigan, USA. He obtained his BS from Manchester College and his PhD in Biophysics from Harvard University. He was a fellow of the Damon Runyon-Walter Winchell Cancer Fund at Stanford University.
References
- AJ Clark, HR Petty, “WO3/Pt nanoparticles promote light-induced lipid peroxidation and lysosomal instability within tumor cells”, Nanotechnology, 27, 075103 (2016). PMID: 26788907. AJ Clark et al., “WO3/Pt nanoparticles are NADPH oxidase biomimetics that mimic effector cells in vitro and in vivo”, Nanotechnology, 27, 065101 (2016). PMID: 26683660. HR Petty, “Could nanoparticles that mimic the NADPH oxidase be used to kill tumor cells?”, Nanomedicine (Lond), 11, 1631–1634 (2016). PMID: 27348694. S Lanone et al., “Comparative toxicity of 24 manufactured nanoparticles in human alveolar epithelial and macrophage cell lines”, Part Fibre Toxicol, 6, 14 (2009). PMID: 19405955. G Hasegawa et al., “Differential genotoxicity of chemical properties and particle size of rare metal and metal oxide nanoparticles”, J Appl Toxicol, 32, 72–80 (2012). PMID: 22162085.